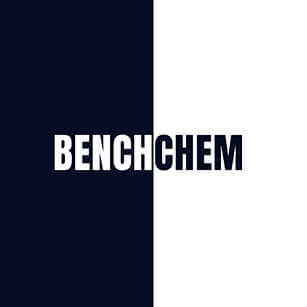
Acarbose Impurity A
- 専門家チームからの見積もりを受け取るには、QUICK INQUIRYをクリックしてください。
- 品質商品を競争力のある価格で提供し、研究に集中できます。
説明
Acarbose Impurity A (CAS: 1013621-79-8) is a structurally related byproduct formed during the microbial fermentation of acarbose, a widely prescribed α-glucosidase inhibitor for type 2 diabetes management . Its molecular formula is C₂₅H₄₃NO₁₈ (molecular weight: 645.6 g/mol), and its IUPAC name is O-4,6-Dideoxy-4-[[(1S,4R,5S,6S)-4,5,6-trihydroxy-3-(hydroxymethyl) cyclohex-2-enyl]amino]-α-D-glucopyranosyl-(1→4)-O-α-D-glucopyranosyl-(1→4)-D-arabino-hex-2-ulopyranose . This impurity arises due to variations in glycosidic linkages and sugar subunit arrangements during biosynthesis. Regulatory agencies like the USFDA and ICH mandate strict control of such impurities (<1.5% threshold) due to their structural similarity to acarbose, which complicates purification and impacts drug safety .
準備方法
Fermentation-Based Generation of Acarbose Impurity A
Microbial Biosynthesis in Actinoplanes sp.
Acarbose and its impurities are predominantly produced via microbial fermentation using Actinoplanes species. During fermentation, Impurity A arises as a side product due to enzymatic modifications of the acarviosyl core. Studies indicate that glycosyltransferases and epimerases catalyze the formation of structural variants, including Impurity A, particularly during the late stages of fermentation . For example, Actinoplanes sp. CKD485-16 produces acarbose titers of 2,300 mg/L alongside 600 mg/L of related impurities under optimized conditions .
Key Fermentation Parameters:
Chromatographic Purification Strategies
Preparative Liquid Chromatography (LC)
The patent CN113670680A outlines a multi-step LC protocol for isolating Impurity A from acarbose-enriched solutions :
-
Initial Concentration :
-
C₁₈ Column Purification :
-
NH₂ Column Refinement :
-
Freeze-Drying :
High-Performance Liquid Chromatography (HPLC)
The USP monograph specifies HPLC-UV methods for impurity profiling, with a focus on resolving Impurity A from acarbose :
CAD outperforms UV in detecting non-chromophoric impurities, eliminating the need for response factors .
Chemical Synthesis and Structural Confirmation
Nuclear Magnetic Resonance (NMR) and Mass Spectrometry
Structural confirmation of Impurity A involves:
-
¹H/¹³C-NMR : Distinct proton signals at δ 5.51 ppm (α-anomeric H) and carbon shifts at δ 89 ppm confirm glycosidic linkages .
-
High-Resolution MS (HRMS) : [M+H]⁺ at m/z 646.2573 (theoretical 646.2553) validates molecular formula C₂₅H₄₃NO₁₈ .
Quality Control and Regulatory Compliance
Pharmacopeial Standards
The European Pharmacopoeia (Ph. Eur.) mandates impurity limits for acarbose formulations :
Impurity | Maximum Allowable Level | Analytical Method |
---|---|---|
A | 0.6% | HPLC-UV or CAD |
C | 1.5% | LC-NMR/MS for structural ID |
Validation Parameters
Method validation follows ICH Q2(R1) guidelines:
化学反応の分析
Types of Reactions: Acarbose Impurity A can undergo various chemical reactions, including oxidation, reduction, and substitution reactions. These reactions can occur during the synthesis, storage, or handling of the compound.
Common Reagents and Conditions:
Oxidation: Common oxidizing agents such as hydrogen peroxide or potassium permanganate can be used to oxidize Impurity A.
Reduction: Reducing agents like sodium borohydride or lithium aluminum hydride can be employed to reduce Impurity A.
Substitution: Nucleophilic substitution reactions can occur with reagents such as sodium hydroxide or other nucleophiles.
Major Products Formed: The major products formed from these reactions depend on the specific conditions and reagents used. For example, oxidation may lead to the formation of carboxylic acids or aldehydes, while reduction may yield alcohols or amines .
科学的研究の応用
Analytical Methods for Detection of Acarbose Impurity A
High-Performance Liquid Chromatography (HPLC)
Recent advancements in HPLC techniques have enhanced the ability to separate acarbose from its impurities, including this compound. One study demonstrated a method achieving a peak-to-valley ratio that allows for effective differentiation between acarbose and its impurities, thus improving the accuracy of acarbose content measurement .
Integrated LC-NMR and LC-MS Approaches
The combination of liquid chromatography with nuclear magnetic resonance (NMR) and mass spectrometry (MS) has been employed to profile impurities in acarbose more effectively. This integrated approach allows for the online separation and structural elucidation of impurities, providing insights into their chemical nature and potential impact on drug quality .
Case Study 1: Production Optimization
A study focused on optimizing production conditions for acarbose highlighted that varying fermentation conditions could significantly influence impurity profiles. By adjusting parameters such as nutrient composition and fermentation time, researchers were able to enhance acarbose yield while simultaneously reducing the formation of impurities, including this compound . This optimization is crucial for industrial applications where cost-effectiveness is paramount.
Case Study 2: Clinical Implications
Acarbose's clinical efficacy can be influenced by its impurity profile. Research indicates that higher levels of impurities may lead to increased gastrointestinal side effects, such as bloating and diarrhea, which are commonly associated with acarbose therapy . Understanding the relationship between impurity levels and patient tolerance can guide clinicians in prescribing practices.
Data Table: Comparison of HPLC Methods for Detecting this compound
Methodology | Key Features | Recovery Rate (%) | RSD (%) |
---|---|---|---|
HPLC with Acetonitrile Buffer | High resolution separation | 99.5 | 0.87 |
Integrated LC-NMR & LC-MS | Structural elucidation of impurities | Not specified | Not specified |
Standard Pharmacopoeia Method | Established method for regulatory compliance | Varies | Varies |
作用機序
The mechanism of action of Acarbose Impurity A is not well-documented, as it is primarily considered an unwanted byproduct. understanding its formation and behavior is crucial for ensuring the purity and efficacy of acarbose. The molecular targets and pathways involved in the formation of Impurity A are related to the biosynthetic pathway of acarbose, which involves the action of various enzymes and intermediates .
類似化合物との比較
Comparison with Structurally Related Impurities
Acarbose fermentation generates multiple impurities, including A, B, C, and D, which differ in glycosidic bonds and sugar subunit composition. Below is a comparative analysis:
Table 1: Structural and Analytical Comparison of Acarbose Impurities
Key Findings:
Structural Differentiation: Impurity A is distinguished by its pentasaccharide backbone and unique arabino-hex-2-ulopyranose subunit, whereas Impurity C is a tetrasaccharide with an aberrant α,α-1,1-trehalose linkage . Impurity C is more challenging to separate from acarbose due to near-identical molecular weight and polarity, necessitating multi-step chromatography .
Impact on Fermentation: Impurity C accumulates during late fermentation stages (e.g., 289 mg/L in Actinoplanes utahensis ZJB-08196 without validamine) and correlates inversely with acarbose yield .
Analytical Challenges :
- Both impurities require LC-MS/MS and NMR for unambiguous identification due to overlapping chromatographic peaks in conventional HPLC .
Comparison with Non-Impurity Analogues
This compound shares functional similarities with other α-glucosidase inhibitors but differs mechanistically:
Table 2: Functional Comparison with Therapeutic Analogues
Key Insights:
- Unlike active analogues (e.g., voglibose, miglitol), Impurity A lacks competitive inhibition of α-glucosidase due to its bulky pentasaccharide structure .
- Structural analogs like validamine (used to suppress Impurity C formation) share cyclitol moieties but act as fermentation modulators rather than direct enzyme inhibitors .
生物活性
Acarbose is an alpha-glucosidase inhibitor used primarily in the management of type 2 diabetes. It functions by delaying the digestion of carbohydrates, thereby reducing postprandial blood glucose levels. However, the presence of impurities, specifically Acarbose Impurity A, can affect its biological activity and therapeutic efficacy. This article delves into the biological activity of this compound, exploring its effects, mechanisms, and implications for clinical use.
Overview of Acarbose and Its Impurities
Acarbose is derived from soil bacteria, particularly from the Actinoplanes genus. It inhibits enzymes responsible for carbohydrate digestion, specifically alpha-glucosidases located in the intestinal brush border. This inhibition leads to a slower absorption of glucose, ultimately aiding in glucose control for diabetic patients .
Impurities in Acarbose:
- This compound : One of the identified impurities that can arise during the production process.
- Other Impurities : Includes Acarbose Impurity B and C, which have been characterized through various analytical methods .
Clinical Studies and Findings
Research has indicated that impurities can affect the pharmacodynamics and pharmacokinetics of drugs. In the case of acarbose:
- Efficacy Variability : Studies have shown that patients receiving acarbose with higher impurity levels may experience less effective glycemic control compared to those receiving purer formulations .
- Adverse Effects : The presence of impurities may also contribute to gastrointestinal side effects commonly associated with acarbose therapy, such as flatulence and diarrhea .
Data Tables
Parameter | Acarbose | This compound |
---|---|---|
Alpha-glucosidase Inhibition | High | Moderate |
Impact on Postprandial Glucose | Significant | Variable |
Gastrointestinal Side Effects | Common | Potentially Increased |
Case Studies
Several case studies have been conducted to assess the impact of impurities on drug efficacy:
- Study on Efficacy Variability :
- Adverse Effect Assessment :
Q & A
Basic Research Questions
Q. What analytical methods are recommended for detecting and quantifying Acarbose Impurity A in pharmaceutical formulations?
Answer: this compound is typically analyzed using hyphenated chromatographic techniques. The European Pharmacopoeia (Ph. Eur.) monograph specifies HPLC-UV methods with specific column parameters (e.g., 4-mm × 625-cm L8 packing, 210-nm detection) for impurity profiling . However, limitations in UV detection for non-chromophoric impurities have led to advancements like HPLC with Charged Aerosol Detection (CAD), which improves sensitivity for impurities lacking strong UV absorption. For structural confirmation, integrated LC-NMR and LC-MS are critical for online separation and elucidation, as demonstrated in studies identifying Impurity A as a pentasaccharide variant differing in sugar subunits .
Q. How should researchers validate analytical methods for this compound quantification?
Answer: Method validation must adhere to ICH guidelines, including parameters such as specificity, accuracy, linearity, and solution stability. For example:
- Specificity : Resolve Impurity A from the acarbose peak, ensuring a valley-to-peak height ratio ≥1.2 .
- Linearity : Establish over a range of 0.1%–5.0% of the API concentration, with R² ≥0.99.
- Accuracy : Spike recovery studies (e.g., 80%–120% of the target limit) using LC-MS/MS or GC-MS/MS for trace-level validation .
Q. What structural characteristics differentiate this compound from the parent compound?
Answer: Structural elucidation via LC-NMR and HRMS reveals that Impurity A (C25H43NO18) is a pentasaccharide with variations in sugar subunit composition and glycosidic linkages compared to acarbose. Key differences include the presence of D-fructose residues and altered cyclitol moieties, confirmed through 2D-NMR experiments .
Advanced Research Questions
Q. How can researchers overcome challenges in structural elucidation of unknown impurities co-eluting with this compound?
Answer: Integrated LC-NMR/MS workflows enable real-time structural analysis. For instance, stop-flow LC-NMR coupled with HRMS can differentiate isobaric impurities by comparing fragmentation patterns and nuclear Overhauser effect (NOE) correlations. This approach was critical in identifying an unknown impurity (C) as a structural analog with additional hydroxyl groups .
Q. What chromatographic columns and conditions optimize impurity profiling for this compound?
Answer:
- Hypercarb Graphite Columns : Provide superior retention for polar impurities using gradient elution (e.g., 1 mL/min flow, 35°C) and CAD detection .
- Amide HILIC Columns : Enhance resolution for hydrophilic impurities under MS-compatible conditions (e.g., Accucore™ 150 Amide HILIC) .
- Comparison : Hypercarb offers stability over APS-2 columns, while Amide HILIC improves peak symmetry for degradation products .
Q. How should researchers address discrepancies in impurity profiles generated by UV vs. CAD detection?
Answer: UV detection may underestimate impurities lacking chromophores (e.g., aliphatic side chains). CAD, being mass-sensitive, detects all non-volatile impurities, including those invisible to UV. For harmonized data, cross-validate methods using spiked samples and orthogonal techniques like LC-MS. For example, CAD identified 12 additional impurities in acarbose batches compared to UV .
Q. What strategies are effective for isolating and characterizing unknown impurities in acarbose batches?
Answer:
- Isolation : Use preparative HPLC with fraction collection, followed by lyophilization to obtain high-purity samples (>95%) .
- Characterization : Combine offline 1H/13C NMR, COSY, and HSQC to assign stereochemistry. HRMS with electrospray ionization (ESI+) confirms molecular formulas .
- Case Study : An unknown impurity in acarbose was identified as a methylated derivative through NOESY correlations and accurate mass (<2 ppm error) .
Q. What regulatory criteria govern the establishment of impurity limits for this compound?
Answer: Per ICH Q3A, impurities ≥0.1% require identification and qualification. Limits for Impurity A are typically set at ≤0.3% (individual) and ≤3.0% (total impurities) based on toxicological thresholds and batch data. Analytical methods must demonstrate sensitivity to 0.05% via signal-to-noise ratios ≥10 .
Q. Methodological Workflow Table
特性
CAS番号 |
1013621-79-8 |
---|---|
分子式 |
C25H43NO18 |
分子量 |
645.62 |
外観 |
White to Off-White Solid |
純度 |
> 95% |
数量 |
Milligrams-Grams |
同義語 |
O-4,6-Dideoxy-4-[[(1S,4R,5S,6S)-4,5,6-trihydroxy-3-(hydroxymethyl)yranosyl-(1?4)-O-a-D-)-D-fructose; Acarbose D-Fructose Impurit |
製品の起源 |
United States |
試験管内研究製品の免責事項と情報
BenchChemで提示されるすべての記事および製品情報は、情報提供を目的としています。BenchChemで購入可能な製品は、生体外研究のために特別に設計されています。生体外研究は、ラテン語の "in glass" に由来し、生物体の外で行われる実験を指します。これらの製品は医薬品または薬として分類されておらず、FDAから任何の医療状態、病気、または疾患の予防、治療、または治癒のために承認されていません。これらの製品を人間または動物に体内に導入する形態は、法律により厳格に禁止されています。これらのガイドラインに従うことは、研究と実験において法的および倫理的な基準の遵守を確実にするために重要です。