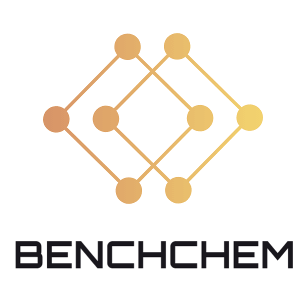
Cefotaxime Impurity D Sodium Salt
- 専門家チームからの見積もりを受け取るには、QUICK INQUIRYをクリックしてください。
- 品質商品を競争力のある価格で提供し、研究に集中できます。
概要
説明
Cefotaxime Impurity D Sodium Salt is a byproduct found in the synthesis of cefotaxime, a third-generation cephalosporin antibiotic. This impurity is formed during the manufacturing process and can affect the purity and efficacy of the final pharmaceutical product. Understanding and controlling the presence of such impurities is crucial for ensuring the safety and effectiveness of cefotaxime.
科学的研究の応用
Cefotaxime Impurity D Sodium Salt has several scientific research applications, including:
Chemistry: Used as a reference standard for analytical methods development and validation.
Biology: Studied for its potential biological activity and interactions with biological molecules.
Medicine: Investigated for its role in the stability and efficacy of cefotaxime formulations.
Industry: Utilized in quality control processes to ensure the purity of cefotaxime sodium.
作用機序
Target of Action
Cefotaxime, the parent compound of Cefotaxime Impurity D Sodium Salt, primarily targets bacterial cell walls. It has a particular potency against Gram-negative bacteria such as Enterobacteriaceae, Haemophilus influenzae, Moraxella (Branhamella) catarrhalis, and Neisseria species . It is also active against some Gram-positive bacteria such as staphylococci and streptococci .
Mode of Action
The bactericidal activity of cefotaxime results from the inhibition of cell wall synthesis via affinity for penicillin-binding proteins (PBPs). Cefotaxime shows high affinity for penicillin-binding proteins in the cell wall including PBP Ib and PBP III . It inhibits bacterial cell wall synthesis by binding to one or more of the PBPs, which in turn inhibits the final transpeptidation step of peptidoglycan synthesis in bacterial cell walls, thus inhibiting cell wall biosynthesis .
Biochemical Pathways
Cefotaxime, like other β-lactam antibiotics, interferes with the final stage of bacterial cell wall synthesis. This interference triggers an autolytic effect in the bacteria, leading to bacterial cell death
Pharmacokinetics
Following intramuscular administration of a single dose of cefotaxime, mean peak serum concentrations are attained within 30 minutes . The main pharmacokinetic parameters of cefotaxime had no significant difference between different dose cohorts and between genders . There was no difference in trough concentrations on days 6, 7, and 8 . The renal cumulative excretion ratios were (51.7 ± 6.2)% for cefotaxime .
Result of Action
The result of cefotaxime’s action is the inhibition of bacterial growth and proliferation, leading to bacterial cell death. This is due to the disruption of the bacterial cell wall synthesis, which is crucial for the bacteria’s survival .
Action Environment
Environmental factors can influence the action of this compound. For instance, the raw materials and preparation process of β-lactam antibiotics may result in polymer impurities during production, transportation, storage, and even use . These impurities, including dimers, trimers, tetramers, and so on, are generated through covalent polymerization between β-lactam antibiotic drug molecules . The stability of this compound under different environmental conditions is a topic that warrants further investigation.
Safety and Hazards
将来の方向性
生化学分析
Biochemical Properties
Cefotaxime Impurity D Sodium Salt plays a significant role in biochemical reactions, particularly in the context of antibiotic activity. It interacts with various enzymes, proteins, and other biomolecules. The compound inhibits bacterial cell wall synthesis by binding to penicillin-binding proteins (PBPs), which are essential for the cross-linking of peptidoglycan layers in bacterial cell walls . This interaction disrupts the integrity of the bacterial cell wall, leading to cell lysis and death. Additionally, this compound may interact with other biomolecules involved in bacterial metabolism and resistance mechanisms.
Cellular Effects
This compound affects various types of cells and cellular processes. In bacterial cells, it inhibits cell wall synthesis, leading to cell death. This compound also influences cell signaling pathways, gene expression, and cellular metabolism. For instance, the inhibition of PBPs by this compound can trigger stress responses in bacteria, leading to changes in gene expression and metabolic adaptations . In mammalian cells, the compound may have limited direct effects, but its presence can influence the overall cellular environment by affecting the microbiome and immune responses.
Molecular Mechanism
The molecular mechanism of action of this compound involves binding interactions with penicillin-binding proteins (PBPs) in bacterial cell walls. By inhibiting these proteins, the compound prevents the cross-linking of peptidoglycan layers, which is crucial for maintaining cell wall integrity . This inhibition leads to the accumulation of cell wall precursors, triggering autolytic enzymes that degrade the cell wall and cause bacterial cell lysis. Additionally, this compound may inhibit other enzymes involved in bacterial metabolism, further contributing to its antibacterial activity.
Temporal Effects in Laboratory Settings
In laboratory settings, the effects of this compound can change over time. The compound’s stability and degradation are critical factors influencing its long-term effects on cellular function. Studies have shown that this compound is relatively stable under controlled conditions, but it can degrade over time, leading to the formation of degradation products . These degradation products may have different biological activities and can influence the overall efficacy and safety of the compound in long-term studies.
Dosage Effects in Animal Models
The effects of this compound vary with different dosages in animal models. At therapeutic doses, the compound effectively inhibits bacterial growth and treats infections. At higher doses, it may cause toxic or adverse effects. Studies in animal models have shown that high doses of this compound can lead to nephrotoxicity, hepatotoxicity, and other adverse effects . It is essential to determine the optimal dosage to balance efficacy and safety in clinical applications.
Metabolic Pathways
This compound is involved in various metabolic pathways. The compound is metabolized primarily in the liver, where it undergoes deacetylation to form desacetylcefotaxime . This metabolite retains antibacterial activity and contributes to the overall efficacy of the compound. Further metabolism of desacetylcefotaxime leads to the formation of inactive metabolites, which are excreted in the urine. The metabolic pathways of this compound are crucial for understanding its pharmacokinetics and optimizing its therapeutic use.
Transport and Distribution
The transport and distribution of this compound within cells and tissues are essential for its biological activity. The compound is transported across cell membranes by specific transporters and binding proteins . Once inside the cells, it can accumulate in specific compartments, such as the cytoplasm and cell wall, where it exerts its antibacterial effects. The distribution of this compound in tissues is influenced by factors such as blood flow, tissue permeability, and binding affinity to cellular components.
Subcellular Localization
This compound exhibits specific subcellular localization, which is crucial for its activity and function. The compound targets the bacterial cell wall, where it binds to penicillin-binding proteins and inhibits cell wall synthesis . Additionally, it may localize to other subcellular compartments involved in bacterial metabolism and resistance mechanisms. Understanding the subcellular localization of this compound is essential for elucidating its mode of action and optimizing its therapeutic use.
準備方法
Synthetic Routes and Reaction Conditions: The preparation of Cefotaxime Impurity D Sodium Salt involves the degradation of cefotaxime sodium. A polymer stock solution of cefotaxime sodium is obtained using a concentrated solution degradation method. The cefotaxime dimer and trimer impurities are separated and purified by preparative reversed-high-performance liquid chromatography (RP-HPLC), and the pure cefotaxime dimer and trimer are obtained by a freeze-drying method .
Industrial Production Methods: In industrial settings, the production of cefotaxime sodium involves multiple steps, including fermentation, chemical synthesis, and purification. During these processes, impurities such as this compound can form. The control of these impurities is achieved through rigorous quality control measures, including chromatographic techniques and spectroscopic analysis .
化学反応の分析
Types of Reactions: Cefotaxime Impurity D Sodium Salt can undergo various chemical reactions, including:
Oxidation: The impurity can be oxidized under specific conditions, leading to the formation of different oxidation products.
Reduction: Reduction reactions can convert the impurity into other reduced forms.
Substitution: Substitution reactions can occur, where functional groups in the impurity are replaced by other groups.
Common Reagents and Conditions:
Oxidizing Agents: Hydrogen peroxide, potassium permanganate.
Reducing Agents: Sodium borohydride, lithium aluminum hydride.
Substitution Reagents: Halogens, alkylating agents.
Major Products Formed: The major products formed from these reactions depend on the specific conditions and reagents used. For example, oxidation can lead to the formation of sulfoxides or sulfones, while reduction can produce alcohols or amines .
類似化合物との比較
Cefotaxime Dimer: Another impurity formed during the synthesis of cefotaxime.
Cefotaxime Trimer: A higher-order polymer impurity.
Cefepime Impurities: Similar impurities found in the synthesis of cefepime, another cephalosporin antibiotic.
Uniqueness: Cefotaxime Impurity D Sodium Salt is unique due to its specific formation pathway and chemical structure. It is formed through the degradation of cefotaxime sodium and can be isolated and characterized using advanced chromatographic and spectroscopic techniques .
特性
{ "Design of the Synthesis Pathway": "The synthesis pathway for Cefotaxime Impurity D Sodium Salt involves the conversion of Cefotaxime Sodium Salt to the desired impurity through a series of chemical reactions.", "Starting Materials": [ "Cefotaxime Sodium Salt", "Sodium Hydroxide", "Hydrochloric Acid", "Methanol", "Water" ], "Reaction": [ "Step 1: Dissolve Cefotaxime Sodium Salt in water and adjust the pH to 7-8 using Sodium Hydroxide.", "Step 2: Add Hydrochloric Acid dropwise to the solution until the pH reaches 2-3.", "Step 3: Extract the solution with Methanol to obtain the impurity.", "Step 4: Convert the impurity to its Sodium Salt form by dissolving it in water and adding Sodium Hydroxide until the pH reaches 7-8.", "Step 5: Filter the solution and dry the resulting Cefotaxime Impurity D Sodium Salt." ] } | |
CAS番号 |
65715-12-0 |
分子式 |
C16H16N5O7S2. Na |
分子量 |
454.46 22.99 |
純度 |
> 95% |
数量 |
Milligrams-Grams |
同義語 |
5-Thia-1-azabicyclo[4.2.0]oct-2-ene-2-carboxylic acid, 3-[(acetyloxy)methyl]-7-[[(2-amino-4-thiazolyl)(methoxyimino)acetyl]amino]-8-oxo-, monosodium salt, [6R-[6α,7β(E)]]- (9CI) |
製品の起源 |
United States |
試験管内研究製品の免責事項と情報
BenchChemで提示されるすべての記事および製品情報は、情報提供を目的としています。BenchChemで購入可能な製品は、生体外研究のために特別に設計されています。生体外研究は、ラテン語の "in glass" に由来し、生物体の外で行われる実験を指します。これらの製品は医薬品または薬として分類されておらず、FDAから任何の医療状態、病気、または疾患の予防、治療、または治癒のために承認されていません。これらの製品を人間または動物に体内に導入する形態は、法律により厳格に禁止されています。これらのガイドラインに従うことは、研究と実験において法的および倫理的な基準の遵守を確実にするために重要です。