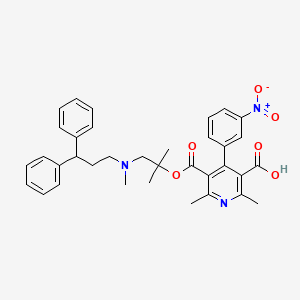
Lercanidipine Impurity B
説明
Lercanidipine Impurity B, also known as 5-(((1-((3,3-diphenylpropyl)(methyl)amino)-2-methylpropan-2-yl)oxy)carbonyl)-2,6-dimethyl-4-(3-nitrophenyl)nicotinic acid, is a chemical impurity associated with the synthesis and degradation of Lercanidipine Hydrochloride. Lercanidipine is a third-generation calcium channel blocker used primarily in the treatment of hypertension. Impurities like this compound are crucial for understanding the stability, efficacy, and safety of the pharmaceutical product .
作用機序
Target of Action
Lercanidipine, the parent compound of Lercanidipine Impurity B, is a calcium channel blocker of the dihydropyridine class . It primarily targets L-type calcium channels in the smooth muscle cells of blood vessels . These channels are responsible for the influx of extracellular calcium across the myocardial and vascular smooth muscle cell membranes .
Mode of Action
Lercanidipine inhibits the influx of extracellular calcium across the myocardial and vascular smooth muscle cell membranes . This inhibition is achieved possibly by deforming the channel, inhibiting ion-control gating mechanisms, and/or interfering with the release of calcium from the sarcoplasmic reticulum . The decrease in intracellular calcium inhibits the contractile processes of the myocardial smooth muscle cells .
Pharmacokinetics
The bioavailability of Lercanidipine is influenced by factors such as polymorphism, particle size, and physiological conditions . For instance, under usual fasted (pH 1.3) and fed (pH 4.9) gastric conditions, the two polymorphs of Lercanidipine have similar bioavailability, regardless of the particle size . At high gastric ph in the fasted state (eg, pH 30), the bioavailability of one form can be considerably lower than that of the other, unless the particle size is less than 20 μm .
Result of Action
The action of Lercanidipine leads to a decrease in blood pressure . This is achieved through the dilation of the coronary and systemic arteries, which in turn decreases total peripheral resistance and systemic blood pressure . The decrease in intracellular calcium also inhibits the contractile processes of the myocardial smooth muscle cells .
Action Environment
The action of Lercanidipine is influenced by environmental factors such as the pH and composition of the buffer media . For example, the solubilities of Lercanidipine polymorphs are strongly dependent on the composition and pH of the buffer media . This highlights the importance of investigating the effect of the buffer nature when evaluating the solubility of ionizable polymorphic substances .
生化学分析
Biochemical Properties
It is known that Lercanidipine, the parent compound, interacts with L-type and T-type calcium channels
Cellular Effects
Lercanidipine has been shown to have effects on various types of cells, particularly in the cardiovascular system where it causes vasodilation
Molecular Mechanism
As an impurity of Lercanidipine, it may share some of the parent compound’s mechanisms, such as binding to and inhibiting L-type and T-type calcium channels
Metabolic Pathways
Lercanidipine is metabolized by the hepatic enzyme cytochrome P450 (CYP) 3A4 , so it’s possible that Lercanidipine Impurity B may be metabolized by similar enzymes
準備方法
Synthetic Routes and Reaction Conditions
The synthesis of Lercanidipine Impurity B involves multiple steps, typically starting from the core structure of Lercanidipine. The process includes the introduction of specific functional groups and the use of various reagents to achieve the desired impurity. Common reagents used in the synthesis include acetonitrile, orthophosphoric acid, and triethylamine. The reaction conditions often involve controlled temperatures and pH adjustments to ensure the correct formation of the impurity .
Industrial Production Methods
Industrial production of this compound follows stringent guidelines to ensure purity and consistency. High-performance liquid chromatography (HPLC) is commonly used to separate and identify the impurity from the main compound. The process involves the use of C18 columns and a mobile phase system containing acetonitrile and aqueous solutions with pH adjustments .
化学反応の分析
Types of Reactions
Lercanidipine Impurity B undergoes various chemical reactions, including:
Oxidation: This reaction involves the addition of oxygen or the removal of hydrogen, often using oxidizing agents.
Reduction: The opposite of oxidation, involving the addition of hydrogen or removal of oxygen.
Substitution: This reaction involves the replacement of one functional group with another.
Common Reagents and Conditions
Common reagents used in these reactions include oxidizing agents like hydrogen peroxide, reducing agents like sodium borohydride, and various acids and bases for substitution reactions. The conditions often involve controlled temperatures and specific pH levels to ensure the desired reaction pathway .
Major Products Formed
The major products formed from these reactions depend on the specific reagents and conditions used. For example, oxidation may lead to the formation of more polar compounds, while reduction may yield less polar products. Substitution reactions can result in a variety of derivatives depending on the substituent introduced .
科学的研究の応用
Lercanidipine Impurity B has several scientific research applications:
Chemistry: Used as a reference standard in analytical chemistry to ensure the purity and stability of Lercanidipine Hydrochloride.
Biology: Studied for its potential biological effects and interactions with various biomolecules.
Medicine: Helps in understanding the pharmacokinetics and pharmacodynamics of Lercanidipine Hydrochloride.
Industry: Used in the quality control and validation processes during the manufacturing of Lercanidipine Hydrochloride
類似化合物との比較
Lercanidipine Impurity B is structurally similar to other impurities and degradation products of Lercanidipine Hydrochloride, such as Impurity 3. These impurities differ in their polarity and acidity, which can be used to fine-tune their retention and separation during analytical procedures. Similar compounds include:
- Nifedipine
- Nitrendipine
- Nimodipine
- Amlodipine These compounds are also calcium channel blockers but differ in their specific chemical structures and pharmacological profiles .
生物活性
Lercanidipine Impurity B (CAS No. 1119226-97-9) is a compound related to lercanidipine, a calcium channel blocker widely used in the treatment of hypertension and angina. While lercanidipine itself has been extensively studied for its pharmacological effects, the biological activity of its impurities, particularly Impurity B, warrants detailed investigation. This article explores the biological activity of this compound, including its mechanism of action, pharmacokinetics, and potential therapeutic implications.
This compound has the molecular formula CHNO and a molecular weight of 595.70 g/mol. It is structurally similar to lercanidipine but differs in polarity and acidity due to its specific functional groups .
Lercanidipine, the parent compound, functions primarily as a dihydropyridine calcium channel blocker . It inhibits the influx of extracellular calcium ions into cardiac and vascular smooth muscle cells by interfering with voltage-dependent calcium channels. This results in decreased intracellular calcium levels, leading to vasodilation and reduced blood pressure .
Pharmacokinetics
The pharmacokinetic profile of this compound has not been extensively documented; however, insights can be drawn from lercanidipine's characteristics:
- Absorption : Lercanidipine is well absorbed in the gastrointestinal tract with peak plasma concentrations reached within 1.5 to 3 hours after administration .
- Distribution : The distribution is rapid and extensive, with over 98% protein binding observed .
- Metabolism : Lercanidipine is primarily metabolized by CYP3A4 into inactive metabolites, with minimal parent drug excreted in urine or feces .
- Elimination : The elimination half-life ranges from 5.8 to 10.5 hours depending on the enantiomer, suggesting a prolonged therapeutic effect despite a relatively short plasma half-life .
Antihypertensive Effects
While direct studies on this compound's antihypertensive properties are lacking, it is plausible that it may exhibit similar effects as lercanidipine due to their structural relationship. Lercanidipine's ability to reduce total peripheral resistance and systemic blood pressure could extend to its impurities under certain conditions.
Anti-inflammatory Activity
Research indicates that lercanidipine possesses anti-inflammatory properties by inhibiting mast cell degranulation and reducing inflammatory responses . If this compound shares this characteristic, it could have implications for treating inflammatory conditions alongside hypertension.
Case Studies
- Animal Models : In studies involving spontaneously hypertensive rats, lercanidipine was shown to decrease renal fibrosis and microalbuminuria independently of blood pressure reduction, suggesting additional protective mechanisms . Similar outcomes could be hypothesized for Impurity B.
- Clinical Observations : Although clinical data on this compound are sparse, observations from lercanidipine treatments indicate potential benefits in endothelial function and vascular health that might also be relevant for its impurities.
特性
IUPAC Name |
5-[1-[3,3-diphenylpropyl(methyl)amino]-2-methylpropan-2-yl]oxycarbonyl-2,6-dimethyl-4-(3-nitrophenyl)pyridine-3-carboxylic acid | |
---|---|---|
Source | PubChem | |
URL | https://pubchem.ncbi.nlm.nih.gov | |
Description | Data deposited in or computed by PubChem | |
InChI |
InChI=1S/C35H37N3O6/c1-23-30(33(39)40)32(27-17-12-18-28(21-27)38(42)43)31(24(2)36-23)34(41)44-35(3,4)22-37(5)20-19-29(25-13-8-6-9-14-25)26-15-10-7-11-16-26/h6-18,21,29H,19-20,22H2,1-5H3,(H,39,40) | |
Source | PubChem | |
URL | https://pubchem.ncbi.nlm.nih.gov | |
Description | Data deposited in or computed by PubChem | |
InChI Key |
XJPFRTCYDMQXMM-UHFFFAOYSA-N | |
Source | PubChem | |
URL | https://pubchem.ncbi.nlm.nih.gov | |
Description | Data deposited in or computed by PubChem | |
Canonical SMILES |
CC1=C(C(=C(C(=N1)C)C(=O)OC(C)(C)CN(C)CCC(C2=CC=CC=C2)C3=CC=CC=C3)C4=CC(=CC=C4)[N+](=O)[O-])C(=O)O | |
Source | PubChem | |
URL | https://pubchem.ncbi.nlm.nih.gov | |
Description | Data deposited in or computed by PubChem | |
Molecular Formula |
C35H37N3O6 | |
Source | PubChem | |
URL | https://pubchem.ncbi.nlm.nih.gov | |
Description | Data deposited in or computed by PubChem | |
Molecular Weight |
595.7 g/mol | |
Source | PubChem | |
URL | https://pubchem.ncbi.nlm.nih.gov | |
Description | Data deposited in or computed by PubChem | |
CAS No. |
1119226-97-9 | |
Record name | 5-[1-[3,3-diphenylpropyl(methyl)amino]-2-methylpropan-2-yl]oxycarbonyl-2,6-dimethyl-4-(3-nitrophenyl)pyridine-3-carboxylic acid | |
Source | European Chemicals Agency (ECHA) | |
URL | https://echa.europa.eu/information-on-chemicals | |
Description | The European Chemicals Agency (ECHA) is an agency of the European Union which is the driving force among regulatory authorities in implementing the EU's groundbreaking chemicals legislation for the benefit of human health and the environment as well as for innovation and competitiveness. | |
Explanation | Use of the information, documents and data from the ECHA website is subject to the terms and conditions of this Legal Notice, and subject to other binding limitations provided for under applicable law, the information, documents and data made available on the ECHA website may be reproduced, distributed and/or used, totally or in part, for non-commercial purposes provided that ECHA is acknowledged as the source: "Source: European Chemicals Agency, http://echa.europa.eu/". Such acknowledgement must be included in each copy of the material. ECHA permits and encourages organisations and individuals to create links to the ECHA website under the following cumulative conditions: Links can only be made to webpages that provide a link to the Legal Notice page. | |
試験管内研究製品の免責事項と情報
BenchChemで提示されるすべての記事および製品情報は、情報提供を目的としています。BenchChemで購入可能な製品は、生体外研究のために特別に設計されています。生体外研究は、ラテン語の "in glass" に由来し、生物体の外で行われる実験を指します。これらの製品は医薬品または薬として分類されておらず、FDAから任何の医療状態、病気、または疾患の予防、治療、または治癒のために承認されていません。これらの製品を人間または動物に体内に導入する形態は、法律により厳格に禁止されています。これらのガイドラインに従うことは、研究と実験において法的および倫理的な基準の遵守を確実にするために重要です。