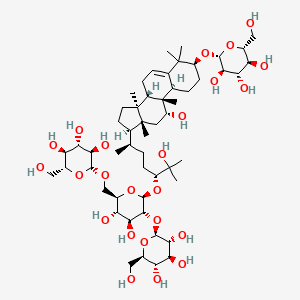
シアメノサイドI
- 専門家チームからの見積もりを受け取るには、QUICK INQUIRYをクリックしてください。
- 品質商品を競争力のある価格で提供し、研究に集中できます。
説明
科学的研究の応用
Shikonin has a wide range of scientific research applications:
Chemistry: Used as a natural dye and in the synthesis of bioactive compounds.
Biology: Studied for its role in cell signaling pathways and gene expression.
Medicine: Investigated for its anticancer, anti-inflammatory, and wound-healing properties
Industry: Utilized in cosmetics, food packaging, and textiles for its antimicrobial and antioxidant properties.
作用機序
Target of Action
- Siamenoside I : The primary targets of Siamenoside I are not clearly defined in the literature .
- Shikonin : Shikonin is a specific inhibitor of pyruvate kinase M2 (PKM2) and can also inhibit TNF-α and NF-κB pathways .
Mode of Action
- Siamenoside I : In rats, Siamenoside I was found to undergo deglycosylation, hydroxylation, dehydrogenation, deoxygenation, isomerization, and glycosylation reactions .
- Shikonin : Shikonin significantly reduces tumor cell viability, proliferation, migration, invasion, and metastasis in both in vivo and in vitro across all breast cancer subtypes . It stimulates immunogenic cell death, resulting in apoptosis and necroptosis .
Biochemical Pathways
- Siamenoside I : The metabolic pathways of Siamenoside I involve deglycosylation, hydroxylation, dehydrogenation, deoxygenation, isomerization, and glycosylation reactions .
- Shikonin : Shikonin affects various cell signaling pathways and possesses antifungal, antibacterial, and allelopathic activity .
Pharmacokinetics
- Siamenoside I : The distributions of Siamenoside I and its metabolites in rat organs were reported, and they were mainly distributed to the intestine, stomach, kidney, and brain .
- Shikonin : Shikonin has an unfavorable oral bioavailability, 64.6% of the binding rate of plasma protein, and enhances some metabolic enzymes, particularly including cytochrome P450 .
Result of Action
- Siamenoside I : Siamenoside I is the sweetest mogroside that has several kinds of bioactivities .
- Shikonin : Shikonin exhibits potent anti-breast cancer properties and shows significant potential for the advancement of immunotherapeutic approaches against breast cancer, as well as enhancing the efficacy of conventional treatment strategies .
Action Environment
生化学分析
Biochemical Properties
In rats, Siamenoside I was found to undergo several biochemical reactions, including deglycosylation, hydroxylation, dehydrogenation, deoxygenation, isomerization, and glycosylation . Among these, deoxygenation, pentahydroxylation, and didehydrogenation were novel metabolic reactions of mogrosides .
Cellular Effects
It is known that Siamenoside I and its metabolites are mainly distributed to the intestine, stomach, kidney, and brain in rats .
Molecular Mechanism
The molecular mechanism of Siamenoside I involves several biochemical reactions. In rats, Siamenoside I was found to undergo deglycosylation, hydroxylation, dehydrogenation, deoxygenation, isomerization, and glycosylation . These reactions suggest that Siamenoside I interacts with various enzymes and other biomolecules in the body.
Temporal Effects in Laboratory Settings
It is known that Siamenoside I and its metabolites are mainly distributed to the intestine, stomach, kidney, and brain in rats .
Dosage Effects in Animal Models
It is known that Siamenoside I and its metabolites are mainly distributed to the intestine, stomach, kidney, and brain in rats .
Metabolic Pathways
In rats, Siamenoside I was found to undergo several metabolic pathways, including deglycosylation, hydroxylation, dehydrogenation, deoxygenation, isomerization, and glycosylation . These reactions suggest that Siamenoside I interacts with various enzymes and other biomolecules in the body.
Transport and Distribution
Siamenoside I and its metabolites are mainly distributed to the intestine, stomach, kidney, and brain in rats . This suggests that Siamenoside I is transported and distributed within cells and tissues.
Subcellular Localization
It is known that Siamenoside I and its metabolites are mainly distributed to the intestine, stomach, kidney, and brain in rats .
準備方法
Synthetic Routes and Reaction Conditions
Shikonin can be synthesized through various methods, including chemical synthesis and biotechnological approaches. One common method involves the use of naphthoquinone intermediates, which undergo a series of reactions such as Friedel-Crafts acylation, reduction, and oxidation to yield shikonin . The reaction conditions typically involve the use of catalysts like aluminum chloride and solvents such as dichloromethane.
Industrial Production Methods
Industrial production of shikonin often relies on the extraction from plant sources. Techniques such as solvent extraction, ultrasonic extraction, and chromatographic separation are commonly used . Recent advancements have also explored the use of genetically engineered biosynthetic pathways to enhance the yield of shikonin from plant cultures .
化学反応の分析
Types of Reactions
Shikonin undergoes various chemical reactions, including:
Oxidation: Shikonin can be oxidized to form shikonin derivatives with different bioactivities.
Reduction: Reduction of shikonin leads to the formation of dihydroshikonin.
Substitution: Shikonin can undergo substitution reactions to form various esterified derivatives.
Common Reagents and Conditions
Oxidation: Common oxidizing agents include potassium permanganate and hydrogen peroxide.
Reduction: Reducing agents such as sodium borohydride are used.
Substitution: Reagents like acyl chlorides and anhydrides are employed under acidic or basic conditions.
Major Products Formed
The major products formed from these reactions include various shikonin derivatives such as acetylshikonin, isobutyrylshikonin, and β-hydroxyisovalerylshikonin .
類似化合物との比較
Shikonin is often compared with other naphthoquinone compounds such as:
Alkannin: Similar in structure and bioactivity, but shikonin has a broader spectrum of pharmacological effects.
Plumbagin: Another naphthoquinone with potent anticancer properties, but shikonin is more effective in wound healing.
Shikonin’s uniqueness lies in its diverse pharmacological activities and its potential for use in various fields, making it a compound of significant interest in scientific research and industrial applications.
特性
CAS番号 |
126105-12-2 |
---|---|
分子式 |
C54H92O24 |
分子量 |
1125.3 g/mol |
IUPAC名 |
(2R,3R,4S,5S,6R)-2-[[(2R,3S,4S,5R,6S)-3,4-dihydroxy-6-[(6R)-2-hydroxy-6-[(3S,8R,9R,10R,11R,13R,14S,17R)-11-hydroxy-4,4,9,13,14-pentamethyl-3-[(2S,3R,4S,5S,6R)-3,4,5-trihydroxy-6-(hydroxymethyl)oxan-2-yl]oxy-2,3,7,8,10,11,12,15,16,17-decahydro-1H-cyclopenta[a]phenanthren-17-yl]-2-methylheptan-3-yl]oxy-5-[(2S,3R,4S,5S,6R)-3,4,5-trihydroxy-6-(hydroxymethyl)oxan-2-yl]oxyoxan-2-yl]methoxy]-6-(hydroxymethyl)oxane-3,4,5-triol |
InChI |
InChI=1S/C54H92O24/c1-22(23-15-16-52(6)30-12-10-24-25(54(30,8)31(58)17-53(23,52)7)11-14-32(50(24,2)3)76-47-43(68)39(64)35(60)27(19-56)73-47)9-13-33(51(4,5)70)77-49-45(78-48-44(69)40(65)36(61)28(20-57)74-48)41(66)37(62)29(75-49)21-71-46-42(67)38(63)34(59)26(18-55)72-46/h10,22-23,25-49,55-70H,9,11-21H2,1-8H3/t22-,23-,25-,26-,27-,28-,29-,30-,31-,32+,33?,34-,35-,36-,37-,38+,39+,40+,41+,42-,43-,44-,45-,46-,47+,48+,49+,52+,53-,54+/m1/s1 |
InChIキー |
XJIPREFALCDWRQ-XZTCQXNCSA-N |
異性体SMILES |
C[C@H](CCC(C(C)(C)O)O[C@H]1[C@@H]([C@H]([C@@H]([C@H](O1)CO[C@H]2[C@@H]([C@H]([C@@H]([C@H](O2)CO)O)O)O)O)O)O[C@H]3[C@@H]([C@H]([C@@H]([C@H](O3)CO)O)O)O)[C@H]4CC[C@@]5([C@@]4(C[C@H]([C@@]6([C@@H]5CC=C7[C@H]6CC[C@@H](C7(C)C)O[C@H]8[C@@H]([C@H]([C@@H]([C@H](O8)CO)O)O)O)C)O)C)C |
正規SMILES |
CC(CCC(C(C)(C)O)OC1C(C(C(C(O1)COC2C(C(C(C(O2)CO)O)O)O)O)O)OC3C(C(C(C(O3)CO)O)O)O)C4CCC5(C4(CC(C6(C5CC=C7C6CCC(C7(C)C)OC8C(C(C(C(O8)CO)O)O)O)C)O)C)C |
製品の起源 |
United States |
Q1: What are the main differences in cytotoxicity between Shikonin and its derivative Acetylshikonin?
A1: Research suggests that while both Shikonin (SH) and Acetylshikonin (ASH) exhibit cytotoxic effects, SH demonstrates a significantly stronger cytotoxic profile compared to ASH. Specifically, the EC50 values (a measure of potency) obtained for SH were approximately two times lower than those observed for ASH when tested against the normal cell line V79. [] This difference in cytotoxicity suggests that ASH might offer a safer profile compared to SH for potential therapeutic applications.
Q2: Does Acetylshikonin exhibit any protective effects against genotoxicity induced by common genotoxic agents?
A2: Studies show that ASH demonstrates promising antigenotoxic potential, particularly against genotoxicity induced by the chemotherapeutic agent Cyclophosphamide (CPA). [] This protective effect was not observed with SH. Interestingly, ASH exhibited a contrasting effect when tested against Ethyl Methanesulfonate (EMS), where it increased EMS-induced genotoxicity while SH showed no significant effect. Both SH and ASH were found to reduce the genotoxicity of Ciprofloxacin (CLFX) in specific experimental settings. These findings highlight the complex interplay between ASH and various genotoxic agents, warranting further investigation to understand its full potential and potential risks.
試験管内研究製品の免責事項と情報
BenchChemで提示されるすべての記事および製品情報は、情報提供を目的としています。BenchChemで購入可能な製品は、生体外研究のために特別に設計されています。生体外研究は、ラテン語の "in glass" に由来し、生物体の外で行われる実験を指します。これらの製品は医薬品または薬として分類されておらず、FDAから任何の医療状態、病気、または疾患の予防、治療、または治癒のために承認されていません。これらの製品を人間または動物に体内に導入する形態は、法律により厳格に禁止されています。これらのガイドラインに従うことは、研究と実験において法的および倫理的な基準の遵守を確実にするために重要です。