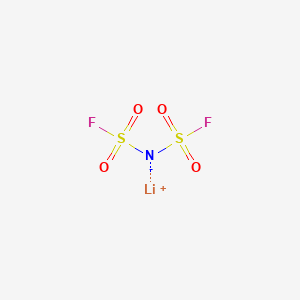
フッ化スルホニルイミドリチウム
- 専門家チームからの見積もりを受け取るには、QUICK INQUIRYをクリックしてください。
- 品質商品を競争力のある価格で提供し、研究に集中できます。
概要
説明
Lithium bis(fluorosulfonyl)imide is a white, powdery lithium salt with the chemical formula F₂LiNO₄S₂. It is commonly used as a high-performance electrolyte material in lithium-ion batteries and supercapacitors due to its excellent electrochemical properties, including high ionic conductivity and thermal stability .
科学的研究の応用
Lithium bis(fluorosulfonyl)imide is widely used in scientific research, particularly in the field of energy storage. It is a key component in the electrolytes of lithium-ion batteries and lithium-ion capacitors . Its superior performance in combination with graphite or silicon anodes makes it a preferred choice for high-performance batteries . Additionally, it is used in the development of non-flammable solvents for lithium-ion batteries, enhancing safety and stability .
作用機序
Target of Action
The primary target of Lithium Bis(fluorosulfonyl)imide is the LiTPA electrode in lithium-ion batteries . The compound plays a crucial role in enhancing battery performance by stabilizing this electrode .
Mode of Action
Lithium Bis(fluorosulfonyl)imide interacts with its target, the LiTPA electrode, by forming a robust solid electrolyte interphase (SEI) layer . This layer is formed through the electrochemical decomposition of Lithium Bis(fluorosulfonyl)imide, which generates insoluble inorganic products like LiF, Li2SO4, and Li3N . These products improve the mechanical integrity and ionic conductivity at the electrode-electrolyte interfaces . This mechanism contrasts with LiPF6-based electrolytes, leading to enhanced cycling stability and longevity of LiTPA electrodes .
Biochemical Pathways
The formation of the SEI layer on the LiTPA electrode involves a series of chemical reactions. The electrochemical decomposition of Lithium Bis(fluorosulfonyl)imide leads to the formation of insoluble inorganic products . These products then accumulate at the electrode-electrolyte interfaces, improving their mechanical integrity and ionic conductivity . This process helps suppress volume changes during cycling .
Pharmacokinetics
While the term “pharmacokinetics” is typically used in the context of drug metabolism, in the case of Lithium Bis(fluorosulfonyl)imide, we can discuss its properties that affect its performance in lithium-ion batteries. Lithium Bis(fluorosulfonyl)imide exhibits high ionic conductivity, excellent thermal stability, and remarkable solubility in non-aqueous solvents . These properties enhance the performance of batteries, particularly in terms of energy density and cycling stability .
Result of Action
The action of Lithium Bis(fluorosulfonyl)imide results in the stabilization of the LiTPA electrode in lithium-ion batteries . This stabilization is achieved through the formation of a robust SEI layer, which improves the mechanical integrity and ionic conductivity at the electrode-electrolyte interfaces . As a result, the cycling stability and longevity of LiTPA electrodes are enhanced .
Action Environment
The action, efficacy, and stability of Lithium Bis(fluorosulfonyl)imide are influenced by various environmental factors. For instance, the compound’s solubility can be affected by the type of solvent used . Additionally, its thermal stability can be influenced by the operating temperature of the battery . Furthermore, the formation of the SEI layer can be affected by the presence of other compounds in the electrolyte .
Safety and Hazards
将来の方向性
準備方法
Synthetic Routes and Reaction Conditions: The synthesis of lithium bis(fluorosulfonyl)imide typically involves a multi-step process. One common method includes the reaction of bis(chlorosulfonyl)imide with lithium fluoride in an anhydrous hydrogen fluoride medium . Another method involves the reaction of difluorosulfimide triethylamine salt with an alkaline lithium salt in the presence of an organic solvent . The reaction is carried out under reduced pressure to remove triethylamine and water, followed by crystallization and drying to obtain the final product .
Industrial Production Methods: Industrial production of lithium bis(fluorosulfonyl)imide often employs a one-pot synthesis method, which simplifies the process and increases yield. This method involves reacting urea, lithium fluoride, and sulfur trioxide in an anhydrous hydrogen fluoride liquid . The process is designed to be economically and environmentally beneficial, with high yield and minimal waste .
化学反応の分析
Types of Reactions: Lithium bis(fluorosulfonyl)imide undergoes various chemical reactions, including hydrolysis, reduction, and decomposition. It is known for its stability against hydrolysis compared to other lithium salts like lithium hexafluorophosphate .
Common Reagents and Conditions: In hydrolysis reactions, lithium bis(fluorosulfonyl)imide reacts with water to form lithium hydroxide and lithium fluoride . In reduction reactions, it can react with lithium metal to form a solid electrolyte interphase (SEI) layer, which is crucial for the performance of lithium-ion batteries .
Major Products Formed: The major products formed from the hydrolysis of lithium bis(fluorosulfonyl)imide include lithium hydroxide and lithium fluoride . In reduction reactions, the formation of a lithium fluoride-rich SEI layer is a significant outcome .
類似化合物との比較
Similar Compounds:
- Lithium bis(trifluoromethanesulfonyl)imide (LiTFSI)
- Lithium hexafluorophosphate (LiPF₆)
- Lithium bis(fluorosulfonyl)amide
Uniqueness: Lithium bis(fluorosulfonyl)imide stands out due to its higher ionic conductivity and better thermal stability compared to lithium hexafluorophosphate . Unlike lithium bis(trifluoromethanesulfonyl)imide, it exhibits lower corrosiveness towards aluminum, making it more suitable for high-voltage applications . Its stability against hydrolysis also makes it a safer alternative for use in lithium-ion batteries .
特性
{ "Design of the Synthesis Pathway": "The synthesis of Lithium bis(fluorosulfonyl)imide can be achieved through a two-step process. The first step involves the reaction of Lithium fluoride with sulfur tetrafluoride to form Lithium fluorosulfonate. The second step involves the reaction of Lithium fluorosulfonate with Lithium imide to form Lithium bis(fluorosulfonyl)imide.", "Starting Materials": [ "Lithium fluoride", "Sulfur tetrafluoride", "Lithium imide" ], "Reaction": [ "Step 1: Lithium fluoride and sulfur tetrafluoride are mixed together in a reaction vessel and heated to a temperature of 150-200°C. The reaction proceeds to form Lithium fluorosulfonate.", "Step 2: Lithium fluorosulfonate is then added to a solution of Lithium imide in anhydrous THF (tetrahydrofuran) at a temperature of -78°C. The reaction mixture is stirred for several hours until Lithium bis(fluorosulfonyl)imide precipitates out as a white solid.", "Step 3: The solid is filtered and washed with THF to remove any impurities. The resulting Lithium bis(fluorosulfonyl)imide can be dried under vacuum and stored for future use." ] } | |
CAS番号 |
171611-11-3 |
分子式 |
F2HLiNO4S2 |
分子量 |
188.1 g/mol |
IUPAC名 |
lithium;bis(fluorosulfonyl)azanide |
InChI |
InChI=1S/F2HNO4S2.Li/c1-8(4,5)3-9(2,6)7;/h3H; |
InChIキー |
PIAMXHUFQAOKRH-UHFFFAOYSA-N |
SMILES |
[Li+].[N-](S(=O)(=O)F)S(=O)(=O)F |
正規SMILES |
[Li].N(S(=O)(=O)F)S(=O)(=O)F |
製品の起源 |
United States |
試験管内研究製品の免責事項と情報
BenchChemで提示されるすべての記事および製品情報は、情報提供を目的としています。BenchChemで購入可能な製品は、生体外研究のために特別に設計されています。生体外研究は、ラテン語の "in glass" に由来し、生物体の外で行われる実験を指します。これらの製品は医薬品または薬として分類されておらず、FDAから任何の医療状態、病気、または疾患の予防、治療、または治癒のために承認されていません。これらの製品を人間または動物に体内に導入する形態は、法律により厳格に禁止されています。これらのガイドラインに従うことは、研究と実験において法的および倫理的な基準の遵守を確実にするために重要です。