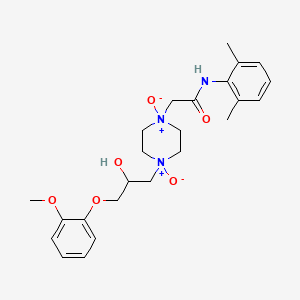
ラノラジンビス(N-オキシド)
説明
Ranolazine Bis(N-Oxide) is a derivative of Ranolazine, a piperazine compound primarily used in the treatment of chronic angina. Ranolazine Bis(N-Oxide) is a modified form of Ranolazine, where the nitrogen atoms in the piperazine ring are oxidized to form N-oxides. This compound is of interest due to its potential enhanced pharmacological properties and unique chemical behavior.
科学的研究の応用
Ranolazine Bis(N-Oxide) has several scientific research applications:
Chemistry: Used as a model compound to study the behavior of N-oxides in various chemical reactions.
Biology: Investigated for its potential biological activities, including anti-inflammatory and anti-cancer properties.
Medicine: Explored for its potential use in treating cardiovascular diseases, similar to Ranolazine, but with potentially enhanced efficacy.
Industry: Used in the development of new pharmaceuticals and as an intermediate in the synthesis of other complex molecules.
作用機序
Target of Action
Ranolazine Bis(N-Oxide) primarily targets the late sodium current (I_Na) in heart muscle cells . This current is present in a variety of voltage-gated sodium channels . The late sodium current plays a crucial role in the regulation of intracellular calcium levels .
Mode of Action
Ranolazine Bis(N-Oxide) interacts with its targets by inhibiting the late sodium current . This inhibition leads to a reduction in intracellular calcium levels . By preventing the late or persistent inward sodium current (I_Na), it causes an increase in intracellular calcium levels to decrease .
Biochemical Pathways
The inhibition of the late sodium current by Ranolazine Bis(N-Oxide) affects several biochemical pathways. It leads to a decrease in intracellular calcium overload, which is believed to be critical to the mechanism of decreased left ventricular relaxation caused by ischemia and reperfusion . Additionally, it prevents fatty acid oxidation and favors glucose utilization, ameliorating the “energy starvation” of the failing heart .
Pharmacokinetics
Ranolazine Bis(N-Oxide) is extensively absorbed after oral administration . It is metabolized in the liver by CYP3A4 and CYP2D6 . The reported clearance rate of orally administered ranolazine is 45 L/h when administered at a dose of 500 mg twice daily . Renal impairment can increase ranolazine serum concentration by 40-50% .
Result of Action
The molecular and cellular effects of Ranolazine Bis(N-Oxide)'s action include a reduction in intracellular calcium levels, leading to reduced tension in the heart wall and reduced oxygen requirements for the muscle . It also inhibits the mitochondrial permeability transition pore (mPTP) opening, cytochrome c release because of cardiolipin peroxidation, and reactive oxygen species formation from mitochondria in heart failure .
Action Environment
Environmental factors such as the presence of other drugs can influence the action, efficacy, and stability of Ranolazine Bis(N-Oxide). For example, co-administration of inhibitors of CYP3A4 and CYP2D6, which are involved in the metabolism of ranolazine, can affect ranolazine’s clearance and increase plasma levels .
生化学分析
Biochemical Properties
Ranolazine Bis(N-Oxide) plays a significant role in biochemical reactions. It interacts with various enzymes and proteins, primarily through its ability to inhibit sodium and potassium ion channel currents . This interaction reduces intracellular calcium overload, which is critical in conditions like ischemia .
Cellular Effects
Ranolazine Bis(N-Oxide) has profound effects on various types of cells and cellular processes. It influences cell function by modulating ion channel activity, which can impact cell signaling pathways, gene expression, and cellular metabolism . For instance, it has been observed to reduce levels of high-sensitive C-reactive protein (CRP) in patients with stable coronary artery disease .
Molecular Mechanism
The molecular mechanism of Ranolazine Bis(N-Oxide) involves its interaction with biomolecules at the molecular level. It exerts its effects primarily through the inhibition of the late phase of the inward sodium current during cardiac repolarization . This action leads to a decrease in intracellular calcium, thereby reducing the tension in the left ventricular diastolic wall and potentially preventing ventricular tachycardia .
Metabolic Pathways
Ranolazine Bis(N-Oxide) is involved in several metabolic pathways. It is known to prevent fatty acid oxidation and favor glucose utilization, thereby ameliorating the “energy starvation” of the failing heart .
Transport and Distribution
Ranolazine Bis(N-Oxide) is transported and distributed within cells and tissues. It is a substrate for P-glycoprotein (P-gp), and inhibitors of P-gp may increase its bioavailability .
準備方法
Synthetic Routes and Reaction Conditions
The synthesis of Ranolazine Bis(N-Oxide) typically involves the oxidation of Ranolazine. One common method is the use of hydrogen peroxide in the presence of a catalyst such as acetic acid. The reaction is carried out under controlled temperature and pH conditions to ensure selective oxidation of the nitrogen atoms in the piperazine ring.
Industrial Production Methods
Industrial production of Ranolazine Bis(N-Oxide) follows similar principles but on a larger scale. The process involves the use of large reactors and precise control of reaction parameters to achieve high yield and purity. The use of continuous flow reactors and advanced purification techniques such as chromatography are common in industrial settings.
化学反応の分析
Types of Reactions
Ranolazine Bis(N-Oxide) undergoes various chemical reactions, including:
Oxidation: Further oxidation can occur under strong oxidizing conditions.
Reduction: The N-oxide groups can be reduced back to the original amine groups using reducing agents like zinc and acetic acid.
Substitution: The N-oxide groups can participate in nucleophilic substitution reactions, leading to the formation of various derivatives.
Common Reagents and Conditions
Oxidation: Hydrogen peroxide, acetic acid.
Reduction: Zinc, acetic acid.
Substitution: Various nucleophiles such as alkyl halides, under basic conditions.
Major Products
Oxidation: Further oxidized products.
Reduction: Ranolazine.
Substitution: Various substituted derivatives of Ranolazine Bis(N-Oxide).
類似化合物との比較
Similar Compounds
Ranolazine: The parent compound, used for treating chronic angina.
Trimetazidine: Another anti-anginal agent with a different mechanism of action.
Ivabradine: A heart rate-reducing agent used in angina treatment.
Uniqueness
Ranolazine Bis(N-Oxide) is unique due to the presence of N-oxide groups, which may enhance its pharmacological properties and chemical reactivity. This makes it a valuable compound for further research and potential therapeutic applications.
特性
IUPAC Name |
N-(2,6-dimethylphenyl)-2-[4-[2-hydroxy-3-(2-methoxyphenoxy)propyl]-1,4-dioxidopiperazine-1,4-diium-1-yl]acetamide | |
---|---|---|
Source | PubChem | |
URL | https://pubchem.ncbi.nlm.nih.gov | |
Description | Data deposited in or computed by PubChem | |
InChI |
InChI=1S/C24H33N3O6/c1-18-7-6-8-19(2)24(18)25-23(29)16-27(31)13-11-26(30,12-14-27)15-20(28)17-33-22-10-5-4-9-21(22)32-3/h4-10,20,28H,11-17H2,1-3H3,(H,25,29) | |
Source | PubChem | |
URL | https://pubchem.ncbi.nlm.nih.gov | |
Description | Data deposited in or computed by PubChem | |
InChI Key |
BIUBGFGNJNWYSV-UHFFFAOYSA-N | |
Source | PubChem | |
URL | https://pubchem.ncbi.nlm.nih.gov | |
Description | Data deposited in or computed by PubChem | |
Canonical SMILES |
CC1=C(C(=CC=C1)C)NC(=O)C[N+]2(CC[N+](CC2)(CC(COC3=CC=CC=C3OC)O)[O-])[O-] | |
Source | PubChem | |
URL | https://pubchem.ncbi.nlm.nih.gov | |
Description | Data deposited in or computed by PubChem | |
Molecular Formula |
C24H33N3O6 | |
Source | PubChem | |
URL | https://pubchem.ncbi.nlm.nih.gov | |
Description | Data deposited in or computed by PubChem | |
DSSTOX Substance ID |
DTXSID00858361 | |
Record name | N-(2,6-Dimethylphenyl)-2-{4-[2-hydroxy-3-(2-methoxyphenoxy)propyl]-1,4-dioxo-1lambda~5~,4lambda~5~-piperazin-1-yl}acetamide | |
Source | EPA DSSTox | |
URL | https://comptox.epa.gov/dashboard/DTXSID00858361 | |
Description | DSSTox provides a high quality public chemistry resource for supporting improved predictive toxicology. | |
Molecular Weight |
459.5 g/mol | |
Source | PubChem | |
URL | https://pubchem.ncbi.nlm.nih.gov | |
Description | Data deposited in or computed by PubChem | |
CAS No. |
1246816-00-1 | |
Record name | Ranolazine bis(N-oxide) | |
Source | ChemIDplus | |
URL | https://pubchem.ncbi.nlm.nih.gov/substance/?source=chemidplus&sourceid=1246816001 | |
Description | ChemIDplus is a free, web search system that provides access to the structure and nomenclature authority files used for the identification of chemical substances cited in National Library of Medicine (NLM) databases, including the TOXNET system. | |
Record name | N-(2,6-Dimethylphenyl)-2-{4-[2-hydroxy-3-(2-methoxyphenoxy)propyl]-1,4-dioxo-1lambda~5~,4lambda~5~-piperazin-1-yl}acetamide | |
Source | EPA DSSTox | |
URL | https://comptox.epa.gov/dashboard/DTXSID00858361 | |
Description | DSSTox provides a high quality public chemistry resource for supporting improved predictive toxicology. | |
Record name | RANOLAZINE BIS(N-OXIDE) | |
Source | FDA Global Substance Registration System (GSRS) | |
URL | https://gsrs.ncats.nih.gov/ginas/app/beta/substances/GF8UVL7D96 | |
Description | The FDA Global Substance Registration System (GSRS) enables the efficient and accurate exchange of information on what substances are in regulated products. Instead of relying on names, which vary across regulatory domains, countries, and regions, the GSRS knowledge base makes it possible for substances to be defined by standardized, scientific descriptions. | |
Explanation | Unless otherwise noted, the contents of the FDA website (www.fda.gov), both text and graphics, are not copyrighted. They are in the public domain and may be republished, reprinted and otherwise used freely by anyone without the need to obtain permission from FDA. Credit to the U.S. Food and Drug Administration as the source is appreciated but not required. | |
試験管内研究製品の免責事項と情報
BenchChemで提示されるすべての記事および製品情報は、情報提供を目的としています。BenchChemで購入可能な製品は、生体外研究のために特別に設計されています。生体外研究は、ラテン語の "in glass" に由来し、生物体の外で行われる実験を指します。これらの製品は医薬品または薬として分類されておらず、FDAから任何の医療状態、病気、または疾患の予防、治療、または治癒のために承認されていません。これらの製品を人間または動物に体内に導入する形態は、法律により厳格に禁止されています。これらのガイドラインに従うことは、研究と実験において法的および倫理的な基準の遵守を確実にするために重要です。