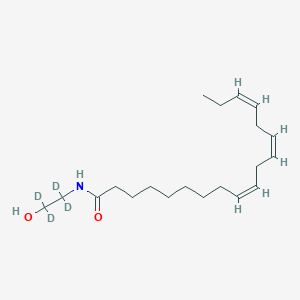
α-リノレンオイルエタノールアミド-d4
概要
説明
α-リノレン酸エタノールアミド-d4: は、アナンダミド(AEA)に見られるアラキドン酸部分をα-リノレン酸に置き換えたエンドカンナビノイドです。 その化学式はC20H35NO2で、分子量は325.5 g/molです 。この化合物は様々な生理学的プロセスに関与していますが、その具体的な機能はまだ解明されていません。
2. 製法
α-リノレン酸エタノールアミド-d4の合成には、重水素標識されたα-リノレン酸をエタノールアミン骨格に組み込むことが含まれます。 これは、GCまたはLC質量分析法を用いたα-リノレン酸エタノールアミドの定量のための内部標準として機能します 。残念ながら、詳細な合成経路や工業生産方法は文献では容易に入手できません。
科学的研究の応用
作用機序
α-リノレン酸エタノールアミド-d4がその効果を発揮する正確なメカニズムは完全には解明されていません。 カンナビノイド受容体に結合し、下流のシグナル伝達経路に影響を与える可能性があります。
6. 類似化合物の比較
α-リノレン酸エタノールアミド-d4は、重水素標識されているためユニークですが、アナンダミド(AEA)や2-アラキドン酸グリセロール(2-AG)などの他のエンドカンナビノイドと類似点を共有しています。これらの化合物は、エンドカンナビノイド系の調節機能に共同で貢献しています。
Safety and Hazards
将来の方向性
生化学分析
Biochemical Properties
Alpha-Linolenoyl Ethanolamide-d4 plays a significant role in biochemical reactions, particularly in the endocannabinoid system. It interacts with cannabinoid receptors, such as CB1 and CB2, which are G-protein-coupled receptors involved in various physiological processes . The interaction between alpha-Linolenoyl Ethanolamide-d4 and these receptors can modulate neurotransmitter release, influencing pain perception, appetite, and mood. Additionally, alpha-Linolenoyl Ethanolamide-d4 may interact with enzymes like fatty acid amide hydrolase (FAAH), which is responsible for the degradation of endocannabinoids .
Cellular Effects
Alpha-Linolenoyl Ethanolamide-d4 affects various cell types and cellular processes. In neuronal cells, it can modulate synaptic transmission by influencing the release of neurotransmitters. This compound also impacts cell signaling pathways, such as the MAPK/ERK pathway, which is involved in cell growth and differentiation . Furthermore, alpha-Linolenoyl Ethanolamide-d4 can alter gene expression by activating transcription factors like CREB, leading to changes in cellular metabolism and function .
Molecular Mechanism
The molecular mechanism of alpha-Linolenoyl Ethanolamide-d4 involves binding interactions with cannabinoid receptors and enzymes. By binding to CB1 and CB2 receptors, it can activate or inhibit downstream signaling pathways, resulting in various physiological effects . Additionally, alpha-Linolenoyl Ethanolamide-d4 can inhibit FAAH, leading to increased levels of endocannabinoids and prolonged signaling . These interactions can result in changes in gene expression and cellular responses.
Temporal Effects in Laboratory Settings
In laboratory settings, the effects of alpha-Linolenoyl Ethanolamide-d4 can change over time. The compound is relatively stable when stored at -20°C, with a shelf life of at least two years . Its stability may decrease under different conditions, leading to degradation and reduced efficacy. Long-term studies have shown that alpha-Linolenoyl Ethanolamide-d4 can have sustained effects on cellular function, particularly in in vitro models .
Dosage Effects in Animal Models
The effects of alpha-Linolenoyl Ethanolamide-d4 vary with different dosages in animal models. At low doses, it can modulate physiological processes without causing significant adverse effects. At higher doses, alpha-Linolenoyl Ethanolamide-d4 may exhibit toxic effects, such as hepatotoxicity and neurotoxicity . Threshold effects have been observed, where a specific dosage is required to achieve the desired physiological response without causing harm .
Metabolic Pathways
Alpha-Linolenoyl Ethanolamide-d4 is involved in various metabolic pathways, particularly those related to lipid metabolism. It interacts with enzymes like FAAH and monoacylglycerol lipase (MAGL), which are involved in the degradation of endocannabinoids . These interactions can affect metabolic flux and alter the levels of metabolites, influencing cellular function and energy homeostasis .
Transport and Distribution
Within cells and tissues, alpha-Linolenoyl Ethanolamide-d4 is transported and distributed through interactions with transporters and binding proteins. It can be taken up by cells via endocytosis or passive diffusion and may bind to intracellular proteins that facilitate its transport to specific cellular compartments . The localization and accumulation of alpha-Linolenoyl Ethanolamide-d4 can influence its activity and function within the cell .
Subcellular Localization
Alpha-Linolenoyl Ethanolamide-d4 is localized in various subcellular compartments, including the plasma membrane, endoplasmic reticulum, and mitochondria . Its activity and function can be affected by post-translational modifications and targeting signals that direct it to specific organelles. These modifications can influence the compound’s stability, interactions with other biomolecules, and overall cellular effects .
化学反応の分析
α-リノレン酸エタノールアミド-d4は、酸化、還元、置換など、様々な反応を起こす可能性があります。 特定の試薬や条件は依然として不足しています。研究者は通常、その反応性を調べるのではなく、分析標準として使用しています。
類似化合物との比較
While α-Linolenoyl Ethanolamide-d4 is unique due to its deuterium labeling, it shares similarities with other endocannabinoids like anandamide (AEA) and 2-arachidonoylglycerol (2-AG). These compounds collectively contribute to the endocannabinoid system’s regulatory functions.
特性
IUPAC Name |
(9Z,12Z,15Z)-N-(1,1,2,2-tetradeuterio-2-hydroxyethyl)octadeca-9,12,15-trienamide | |
---|---|---|
Source | PubChem | |
URL | https://pubchem.ncbi.nlm.nih.gov | |
Description | Data deposited in or computed by PubChem | |
InChI |
InChI=1S/C20H35NO2/c1-2-3-4-5-6-7-8-9-10-11-12-13-14-15-16-17-20(23)21-18-19-22/h3-4,6-7,9-10,22H,2,5,8,11-19H2,1H3,(H,21,23)/b4-3-,7-6-,10-9-/i18D2,19D2 | |
Source | PubChem | |
URL | https://pubchem.ncbi.nlm.nih.gov | |
Description | Data deposited in or computed by PubChem | |
InChI Key |
HBJXRRXWHSHZPU-BBVLJAKGSA-N | |
Source | PubChem | |
URL | https://pubchem.ncbi.nlm.nih.gov | |
Description | Data deposited in or computed by PubChem | |
Canonical SMILES |
CCC=CCC=CCC=CCCCCCCCC(=O)NCCO | |
Source | PubChem | |
URL | https://pubchem.ncbi.nlm.nih.gov | |
Description | Data deposited in or computed by PubChem | |
Isomeric SMILES |
[2H]C([2H])(C([2H])([2H])O)NC(=O)CCCCCCC/C=C\C/C=C\C/C=C\CC | |
Source | PubChem | |
URL | https://pubchem.ncbi.nlm.nih.gov | |
Description | Data deposited in or computed by PubChem | |
Molecular Formula |
C20H35NO2 | |
Source | PubChem | |
URL | https://pubchem.ncbi.nlm.nih.gov | |
Description | Data deposited in or computed by PubChem | |
Molecular Weight |
325.5 g/mol | |
Source | PubChem | |
URL | https://pubchem.ncbi.nlm.nih.gov | |
Description | Data deposited in or computed by PubChem | |
Retrosynthesis Analysis
AI-Powered Synthesis Planning: Our tool employs the Template_relevance Pistachio, Template_relevance Bkms_metabolic, Template_relevance Pistachio_ringbreaker, Template_relevance Reaxys, Template_relevance Reaxys_biocatalysis model, leveraging a vast database of chemical reactions to predict feasible synthetic routes.
One-Step Synthesis Focus: Specifically designed for one-step synthesis, it provides concise and direct routes for your target compounds, streamlining the synthesis process.
Accurate Predictions: Utilizing the extensive PISTACHIO, BKMS_METABOLIC, PISTACHIO_RINGBREAKER, REAXYS, REAXYS_BIOCATALYSIS database, our tool offers high-accuracy predictions, reflecting the latest in chemical research and data.
Strategy Settings
Precursor scoring | Relevance Heuristic |
---|---|
Min. plausibility | 0.01 |
Model | Template_relevance |
Template Set | Pistachio/Bkms_metabolic/Pistachio_ringbreaker/Reaxys/Reaxys_biocatalysis |
Top-N result to add to graph | 6 |
Feasible Synthetic Routes
試験管内研究製品の免責事項と情報
BenchChemで提示されるすべての記事および製品情報は、情報提供を目的としています。BenchChemで購入可能な製品は、生体外研究のために特別に設計されています。生体外研究は、ラテン語の "in glass" に由来し、生物体の外で行われる実験を指します。これらの製品は医薬品または薬として分類されておらず、FDAから任何の医療状態、病気、または疾患の予防、治療、または治癒のために承認されていません。これらの製品を人間または動物に体内に導入する形態は、法律により厳格に禁止されています。これらのガイドラインに従うことは、研究と実験において法的および倫理的な基準の遵守を確実にするために重要です。