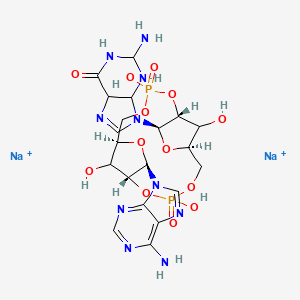
2'2'-cGAMP
- 専門家チームからの見積もりを受け取るには、QUICK INQUIRYをクリックしてください。
- 品質商品を競争力のある価格で提供し、研究に集中できます。
説明
2’2’-cGAMP is a noncanonical cyclic dinucleotide that activates the innate human immune STING .
Synthesis Analysis
The synthesis of 2’2’-cGAMP is catalyzed by the enzyme cyclic GMP-AMP synthase (cGAS). This enzyme is activated upon binding to double-stranded DNA (dsDNA) in the cytosol. The activation of cGAS leads to the synthesis of 2’3’-cGAMP, using Mg²⁺ as the metal cofactor that catalyzes two nucleotidyl-transferring reactions . Structural analysis reveals that Mn²⁺-activated cGAS undergoes globally similar conformational changes to DNA-activated cGAS but forms a unique η1 helix to widen the catalytic pocket, allowing substrate entry and cGAMP synthesis .
Molecular Structure Analysis
The molecular formula of 2’2’-cGAMP is C20H28N10Na2O13P2+2 . The molecular weight is 724.4 g/mol . The structure of 2’2’-cGAMP includes two nucleotides, guanosine and adenosine, linked via two phosphodiester linkages .
Chemical Reactions Analysis
The chemical reactions involving 2’2’-cGAMP are primarily related to its synthesis by cGAS. The enzyme cGAS is activated upon binding to dsDNA, leading to the synthesis of 2’3’-cGAMP . The Mn²⁺-activated cGAS undergoes conformational changes to allow substrate entry and cGAMP synthesis .
Physical And Chemical Properties Analysis
The physical and chemical properties of 2’2’-cGAMP include a molecular weight of 724.4 g/mol, a hydrogen bond donor count of 8, and a hydrogen bond acceptor count of 20 .
科学的研究の応用
がん治療のための免疫療法
2'2'-cGAMPは、インターフェロン遺伝子(STING)経路の刺激因子において重要な役割を果たしており、がん治療のための潜在的な免疫療法として研究されています . STING経路は、哺乳類細胞の細胞質における二本鎖DNA(dsDNA)の存在によって活性化されます。これは、細胞損傷またはがんを示唆しています .
全細胞生物触媒によるcGAMPの製造
This compoundの製造は、マウスの環状グアノシンモノホスフェート-アデノシンモノホスフェートシンターゼ(mcGAS)を利用した全細胞生物触媒によって達成できます . この方法は、有機溶媒を必要とせず、cGAMPの製造のための効率的で環境に優しいプロセスを提供します .
マルチ酵素カスケード反応
This compoundは、マルチ酵素カスケード反応によって合成することができます . これらの反応は、反応中間体の精製、不安定な中間体の取り扱い、および結合平衡による不利な熱力学に対処することなく、複雑な分子を合成する利点があります .
GTPおよびアデノシンからのthis compoundの合成
確立された酵素カスケードは、生物触媒によるワンポット反応で、GTPと安価なアデノシン、およびポリリン酸からthis compoundを合成することを可能にします . これは、医薬品関連製品の合成におけるマルチ酵素カスケードの性能能力を示しています .
研究グレードのcGAMPの製造
研究規模では、標準的な20 mLの培養から、細胞を収穫してから1日以内に2 mgのcGAMPを製造することができます . この研究グレードのプロセスは、1 mgのcGAMPの市場価格と比較して有利です<a aria-label="1: At the research scale, it is possible to produce 2 mg of cGAMP from a standard 20 mL culture within 1 day of harvesting the cells1" data-citationid="e1f87f54-ba5f-b70b-e97c-0d6f88f104b
作用機序
Target of Action
The primary target of 2’2’-cGAMP is the Stimulator of Interferon Genes (STING) . STING is a membrane protein that plays a key role in innate immunity . It is activated by binding to 2’2’-cGAMP, leading to a series of immune responses .
Pharmacokinetics
It is known that the compound has low bioavailability due to its poor stability in serum . Therefore, it is necessary to design new compounds or drug delivery systems with improved pharmacokinetics to enhance cellular uptake, improve cytosolic delivery, and increase the compound’s bioavailability .
Action Environment
The action of 2’2’-cGAMP is influenced by various environmental factors. For instance, the presence of cytosolic DNA, which can be indicative of microbial infection or cellular damage, triggers the synthesis of 2’2’-cGAMP
Safety and Hazards
将来の方向性
The cGAS-STING pathway, which 2’2’-cGAMP activates, has been identified as a potential therapeutic target in various diseases, including inflammatory diseases and cancer . Future research may focus on developing therapeutic strategies that harness the cGAMP-mediated antitumor response for cancer therapy .
生化学分析
Biochemical Properties
2’2’-cGAMP interacts with a variety of enzymes and proteins within the cell. The primary interaction is with the Stimulator of Interferon Genes (STING) receptor . Upon binding to STING, 2’2’-cGAMP triggers a conformational change in the receptor, leading to its activation . This activation initiates a cascade of downstream signaling events, including the activation of Interferon Regulatory Factor 3 (IRF3) and Nuclear Factor kappa-B (NF-κB), which ultimately results in the production of type I interferons .
Cellular Effects
The effects of 2’2’-cGAMP on cellular processes are vast and varied. It influences cell function by impacting cell signaling pathways, gene expression, and cellular metabolism . For instance, the activation of the cGAS-STING pathway by 2’2’-cGAMP can lead to the production of type I interferons, which play a crucial role in innate immunity . Additionally, 2’2’-cGAMP can trigger sterile inflammation in response to cellular stress, such as senescence and mitochondrial stress .
Molecular Mechanism
The molecular mechanism of 2’2’-cGAMP involves its synthesis by cGAS in response to the presence of double-stranded DNA . Once synthesized, 2’2’-cGAMP binds to the STING receptor, triggering a conformational change and subsequent activation . This activation leads to a cascade of downstream signaling events, ultimately resulting in the production of type I interferons .
Dosage Effects in Animal Models
The effects of 2’2’-cGAMP can vary with different dosages in animal models . For instance, a single intranasal dose of a nanoparticle formulation of 2’2’-cGAMP was shown to protect against pathogenic strains of SARS-CoV-2 in hamsters .
Metabolic Pathways
2’2’-cGAMP is involved in several metabolic pathways. It is synthesized by cGAS using ATP and GTP . The cGAS-STING pathway, which 2’2’-cGAMP activates, plays a crucial role in regulating immunity and the host damage repair system .
Transport and Distribution
2’2’-cGAMP is transported and distributed within cells and tissues through its interaction with the STING receptor . Upon binding to STING, 2’2’-cGAMP triggers a conformational change in the receptor, leading to its activation and subsequent translocation from the endoplasmic reticulum to the Golgi apparatus .
Subcellular Localization
The subcellular localization of 2’2’-cGAMP is primarily in the cytoplasm, where it is synthesized by cGAS in response to the presence of double-stranded DNA . Upon binding to the STING receptor, 2’2’-cGAMP triggers the receptor’s translocation from the endoplasmic reticulum to the Golgi apparatus .
特性
IUPAC Name |
disodium;2-amino-9-[(1R,6R,8R,9R,14R,16R)-16-(6-aminopurin-9-yl)-3,11,17,18-tetrahydroxy-3,11-dioxo-2,4,7,10,12,15-hexaoxa-3λ5,11λ5-diphosphatricyclo[12.2.1.16,9]octadecan-8-yl]-2,3,4,5-tetrahydro-1H-purin-6-one |
Source
|
---|---|---|
Source | PubChem | |
URL | https://pubchem.ncbi.nlm.nih.gov | |
Description | Data deposited in or computed by PubChem | |
InChI |
InChI=1S/C20H28N10O13P2.2Na/c21-14-8-15(24-3-23-14)29(4-25-8)18-12-10(31)6(40-18)1-38-45(36,37)43-13-11(32)7(2-39-44(34,35)42-12)41-19(13)30-5-26-9-16(30)27-20(22)28-17(9)33;;/h3-7,9-13,16,18-20,27,31-32H,1-2,22H2,(H,28,33)(H,34,35)(H,36,37)(H2,21,23,24);;/q;2*+1/t6-,7-,9?,10?,11?,12-,13-,16?,18-,19-,20?;;/m1../s1 |
Source
|
Source | PubChem | |
URL | https://pubchem.ncbi.nlm.nih.gov | |
Description | Data deposited in or computed by PubChem | |
InChI Key |
LDAANMXFZNIJRX-VVHLBRQOSA-N |
Source
|
Source | PubChem | |
URL | https://pubchem.ncbi.nlm.nih.gov | |
Description | Data deposited in or computed by PubChem | |
Canonical SMILES |
C1C2C(C(C(O2)N3C=NC4C3NC(NC4=O)N)OP(=O)(OCC5C(C(C(O5)N6C=NC7=C(N=CN=C76)N)OP(=O)(O1)O)O)O)O.[Na+].[Na+] |
Source
|
Source | PubChem | |
URL | https://pubchem.ncbi.nlm.nih.gov | |
Description | Data deposited in or computed by PubChem | |
Isomeric SMILES |
C1[C@@H]2C([C@H]([C@@H](O2)N3C=NC4C3NC(NC4=O)N)OP(=O)(OC[C@@H]5C([C@H]([C@@H](O5)N6C=NC7=C(N=CN=C76)N)OP(=O)(O1)O)O)O)O.[Na+].[Na+] |
Source
|
Source | PubChem | |
URL | https://pubchem.ncbi.nlm.nih.gov | |
Description | Data deposited in or computed by PubChem | |
Molecular Formula |
C20H28N10Na2O13P2+2 |
Source
|
Source | PubChem | |
URL | https://pubchem.ncbi.nlm.nih.gov | |
Description | Data deposited in or computed by PubChem | |
Molecular Weight |
724.4 g/mol |
Source
|
Source | PubChem | |
URL | https://pubchem.ncbi.nlm.nih.gov | |
Description | Data deposited in or computed by PubChem | |
Q & A
Q1: What is the significance of cyclic GMP-AMP (cGAMP) and its analogs in mammals?
A1: Cyclic GMP-AMP synthase (cGAS) synthesizes cGAMP, including its isomer 2'2'-cGAMP, upon detecting cytosolic DNA. [, ] This makes cGAMP and its analogs crucial signaling molecules in the mammalian innate immune response. They are currently being investigated for their potential in pharmaceutical formulations, including vaccine adjuvants, drug screening assays, therapeutic interventions, and diagnostic tools. [, ]
Q2: Can you elaborate on the applications of this compound in drug discovery?
A2: While the provided abstracts primarily highlight cGAMP as a family, this compound, being an isomer, holds potential for similar applications. Research suggests that targeting the cGAS-cGAMP pathway could be valuable for developing:
試験管内研究製品の免責事項と情報
BenchChemで提示されるすべての記事および製品情報は、情報提供を目的としています。BenchChemで購入可能な製品は、生体外研究のために特別に設計されています。生体外研究は、ラテン語の "in glass" に由来し、生物体の外で行われる実験を指します。これらの製品は医薬品または薬として分類されておらず、FDAから任何の医療状態、病気、または疾患の予防、治療、または治癒のために承認されていません。これらの製品を人間または動物に体内に導入する形態は、法律により厳格に禁止されています。これらのガイドラインに従うことは、研究と実験において法的および倫理的な基準の遵守を確実にするために重要です。