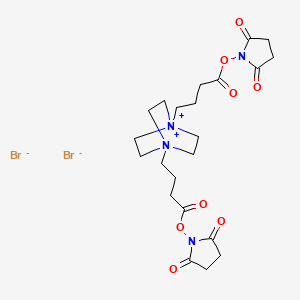
DC4 Crosslinker
- 専門家チームからの見積もりを受け取るには、QUICK INQUIRYをクリックしてください。
- 品質商品を競争力のある価格で提供し、研究に集中できます。
説明
DC4 クロスリンカーは、正式には1,4-ビス[4-[(2,5-ジオキソ-1-ピロリジニル)オキシ]-4-オキソブチル]-1,4-ジアゾニアビシクロ[2.2.2]オクタン、ジブロミドとして知られており、質量分析法で切断可能な架橋試薬です。 これは主に、衝突誘起解離またはインソース崩壊によって架橋ペプチドを構成ペプチドに断片化する能力により、タンパク質相互作用研究で使用されます .
作用機序
DC4 クロスリンカーは、タンパク質間に共有結合を形成することによって作用し、タンパク質-タンパク質相互作用を安定化させます。この化合物は、2つの本質的な正電荷を含み、これにより架橋されたペプチドは、衝突誘起解離またはインソース崩壊によって構成ペプチドに断片化されます。 この断片化により、架橋されたペプチドを、定義された質量によって分離されたイオンのペアとして識別することができます . DC4 クロスリンカーの分子標的は主にタンパク質であり、関与する経路にはタンパク質-タンパク質相互作用経路が含まれます .
類似化合物の比較
類似化合物
ビス(スルホスクシンイミジル)スベレート(BS3): タンパク質相互作用研究で使用される別の架橋試薬。
ジスクシンイミジルスベレート(DSS): タンパク質複合体を安定化させるために一般的に使用される架橋剤。
スルホ-EGS(エチレングリコールビス(スルホスクシンイミジルスクシネート)): さまざまな生化学的応用で使用される水溶性架橋剤.
DC4 クロスリンカーの独自性
DC4 クロスリンカーは、質量分析法で切断可能な性質により、断片化によって架橋されたペプチドを特定できるため、独特です。この特徴は、この機能を持たない他の架橋剤とは異なります。 さらに、本質的な正電荷の存在は、反応性と安定性を向上させ、タンパク質相互作用研究における貴重なツールとなっています .
生化学分析
Biochemical Properties
DC4 Crosslinker plays a significant role in biochemical reactions. It contains two intrinsic positive charges, which allow cross-linked peptides to fragment into their component peptides by collision-induced dissociation or in-source decay . Initial fragmentation events result in cleavage on either side of the positive charges so that cross-linked peptides can be identified as pairs of ions separated by defined masses . Additionally, these two intact peptide fragments can be further fragmented to yield a series of b- and y- ions for peptide identification .
Cellular Effects
The this compound’s effects on cells and cellular processes are primarily through its role in protein interactions. By facilitating the cross-linking of peptides, it can influence cell function, including impacts on cell signaling pathways, gene expression, and cellular metabolism
Molecular Mechanism
The this compound exerts its effects at the molecular level through its unique structure and properties. Its two intrinsic positive charges allow it to participate in the fragmentation of cross-linked peptides . This process results in the cleavage on either side of the positive charges, enabling the identification of cross-linked peptides as pairs of ions separated by defined masses . This mechanism allows for the robust determination of the structures of the component peptides .
Temporal Effects in Laboratory Settings
In laboratory settings, the effects of the this compound can change over time. It is stable to storage, highly reactive, highly soluble, and quite labile to collision-induced dissociation . Over time, this can result in changes in the product’s stability, degradation, and long-term effects on cellular function in in vitro or in vivo studies.
Metabolic Pathways
The this compound is involved in metabolic pathways related to protein interactions It interacts with enzymes and other biomolecules during these processes
準備方法
合成経路と反応条件
DC4 クロスリンカーの合成には、環状アミンと特定の試薬を反応させて目的の架橋構造を形成させることが含まれます。このプロセスには通常、以下の手順が含まれます。
コア構造の形成: クロスリンカーのコア構造は、環状アミンと適切な試薬を制御された条件下で反応させることで合成されます。
官能基の添加: 2,5-ジオキソ-1-ピロリジニルなどの官能基が、コア構造に添加されて、反応性と安定性を向上させます。
工業生産方法
DC4 クロスリンカーの工業生産は、同様の合成経路に従いますが、より大規模に行われます。このプロセスには以下が含まれます。
バルク合成: コア構造の大量が、工業用反応器で合成されます。
官能基化: 官能基が、コア構造にバルクで添加されます。
精製と品質管理: 最終製品は精製され、一貫性と純度を保証するために厳格な品質管理対策が施されます.
化学反応の分析
反応の種類
DC4 クロスリンカーは、以下を含むいくつかの種類の化学反応を受けます。
置換反応: この化合物は、官能基が他の基に置き換わる置換反応に参加できます。
一般的な試薬と条件
DC4 クロスリンカーとの反応で使用される一般的な試薬には以下が含まれます。
酸化剤: 過酸化水素や過マンガン酸カリウムなど。
還元剤: 水素化ホウ素ナトリウムや水素化アルミニウムリチウムなど。
形成される主な生成物
DC4 クロスリンカーを含む反応から形成される主な生成物は、使用される特定の反応条件と試薬によって異なります。 たとえば、酸化反応は酸化された誘導体を生成する可能性があり、置換反応は置換されたクロスリンカー化合物を生成する可能性があります .
科学研究への応用
DC4 クロスリンカーは、以下を含む幅広い科学研究への応用を有しています。
科学的研究の応用
DC4 Crosslinker has a wide range of scientific research applications, including:
Protein interaction studies: It is used to map protein interactions and their dynamics by crosslinking proteins and analyzing the resulting complexes using mass spectrometry.
Structural biology: The compound helps in determining the structure of protein complexes by stabilizing interactions for analysis.
Drug discovery: It aids in identifying potential drug targets by mapping protein-protein interactions.
Biotechnology: The compound is used in the development of biotechnological applications, such as enzyme immobilization and biosensor development.
類似化合物との比較
Similar Compounds
Bis(sulfosuccinimidyl)suberate (BS3): Another crosslinking reagent used in protein interaction studies.
Disuccinimidyl suberate (DSS): A commonly used crosslinker for stabilizing protein complexes.
Sulfo-EGS (ethylene glycol bis(sulfosuccinimidylsuccinate)): A water-soluble crosslinker used in various biochemical applications.
Uniqueness of DC4 Crosslinker
This compound is unique due to its mass spectrometry-cleavable nature, which allows for the identification of crosslinked peptides by fragmentation. This feature distinguishes it from other crosslinkers that do not have this capability. Additionally, the presence of intrinsic positive charges enhances its reactivity and stability, making it a valuable tool in protein interaction studies .
特性
IUPAC Name |
(2,5-dioxopyrrolidin-1-yl) 4-[4-[4-(2,5-dioxopyrrolidin-1-yl)oxy-4-oxobutyl]-1,4-diazoniabicyclo[2.2.2]octan-1-yl]butanoate;dibromide |
Source
|
---|---|---|
Source | PubChem | |
URL | https://pubchem.ncbi.nlm.nih.gov | |
Description | Data deposited in or computed by PubChem | |
InChI |
InChI=1S/C22H32N4O8.2BrH/c27-17-5-6-18(28)23(17)33-21(31)3-1-9-25-11-14-26(15-12-25,16-13-25)10-2-4-22(32)34-24-19(29)7-8-20(24)30;;/h1-16H2;2*1H/q+2;;/p-2 |
Source
|
Source | PubChem | |
URL | https://pubchem.ncbi.nlm.nih.gov | |
Description | Data deposited in or computed by PubChem | |
InChI Key |
AJWAJFDEYIAPKX-UHFFFAOYSA-L |
Source
|
Source | PubChem | |
URL | https://pubchem.ncbi.nlm.nih.gov | |
Description | Data deposited in or computed by PubChem | |
Canonical SMILES |
C1CC(=O)N(C1=O)OC(=O)CCC[N+]23CC[N+](CC2)(CC3)CCCC(=O)ON4C(=O)CCC4=O.[Br-].[Br-] |
Source
|
Source | PubChem | |
URL | https://pubchem.ncbi.nlm.nih.gov | |
Description | Data deposited in or computed by PubChem | |
Molecular Formula |
C22H32Br2N4O8 |
Source
|
Source | PubChem | |
URL | https://pubchem.ncbi.nlm.nih.gov | |
Description | Data deposited in or computed by PubChem | |
Molecular Weight |
640.3 g/mol |
Source
|
Source | PubChem | |
URL | https://pubchem.ncbi.nlm.nih.gov | |
Description | Data deposited in or computed by PubChem | |
Q1: What is unique about the DC4 crosslinker compared to other crosslinkers used in studying protein interactions?
A1: The this compound belongs to a class of quaternary diamines designed to be cleavable in mass spectrometry. [] This feature is crucial because it allows researchers to identify crosslinked peptides more easily. Traditional crosslinkers often create complex data that is challenging to analyze by mass spectrometry. The cleavable nature of DC4 simplifies this analysis, making it a valuable tool for studying protein interactions.
試験管内研究製品の免責事項と情報
BenchChemで提示されるすべての記事および製品情報は、情報提供を目的としています。BenchChemで購入可能な製品は、生体外研究のために特別に設計されています。生体外研究は、ラテン語の "in glass" に由来し、生物体の外で行われる実験を指します。これらの製品は医薬品または薬として分類されておらず、FDAから任何の医療状態、病気、または疾患の予防、治療、または治癒のために承認されていません。これらの製品を人間または動物に体内に導入する形態は、法律により厳格に禁止されています。これらのガイドラインに従うことは、研究と実験において法的および倫理的な基準の遵守を確実にするために重要です。