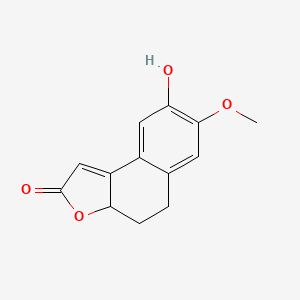
ムセラクトン
- 専門家チームからの見積もりを受け取るには、QUICK INQUIRYをクリックしてください。
- 品質商品を競争力のある価格で提供し、研究に集中できます。
説明
科学的研究の応用
Chemistry: It serves as a model compound for studying lactone chemistry and reaction mechanisms.
作用機序
Target of Action
It was isolated from the aerial parts of Musella lasiocarpa , a plant native to southwestern China
Biochemical Pathways
It’s suggested that diarylheptanoids (dhs), known from many plant families, serve as precursors in the biosynthesis of phenylphenalenones (pps), which are complex phenolic natural products
Pharmacokinetics
As a new compound, its bioavailability and how it’s absorbed, distributed, metabolized, and excreted in the body are subjects of ongoing research .
Result of Action
It’s been observed that musellactone showed different effects against five bacteria strains
Action Environment
The action environment of Musellactone is likely influenced by various factors. For instance, Musellactone is a major component of Musella lasiocarpa seed coats at middle and late developmental stages . This suggests that its production and action might be influenced by the plant’s developmental stage and environmental conditions.
準備方法
Synthetic Routes and Reaction Conditions: Musellactone can be synthesized through various organic reactions involving the precursor compounds found in Musella lasiocarpa. The preparation method involves the extraction of the compound from the plant material followed by purification using chromatographic techniques .
Industrial Production Methods: Industrial production of musellactone is not well-documented, but it typically involves large-scale extraction from Musella lasiocarpa. The process includes harvesting the plant, drying, and extracting the compound using solvents such as ethanol or methanol. The extract is then subjected to purification processes to isolate musellactone .
化学反応の分析
Types of Reactions: Musellactone undergoes various chemical reactions, including:
Oxidation: Musellactone can be oxidized to form different derivatives.
Reduction: Reduction reactions can modify the lactone ring structure.
Substitution: Substitution reactions can introduce new functional groups into the musellactone molecule.
Common Reagents and Conditions:
Oxidation: Common oxidizing agents include potassium permanganate and chromium trioxide.
Reduction: Reducing agents such as lithium aluminum hydride and sodium borohydride are used.
Substitution: Reagents like halogens and alkylating agents are employed for substitution reactions.
Major Products: The major products formed from these reactions include various oxidized, reduced, and substituted derivatives of musellactone, each with distinct chemical properties and potential biological activities .
類似化合物との比較
Musellactone is structurally similar to other lactones and phenylphenalenones found in Musella lasiocarpa. Similar compounds include:
- Musellarin A
- Musellarin B
- Musellarin C
- Neuchromenin
Uniqueness: Musellactone is unique due to its specific lactone ring structure and the presence of distinct functional groups that confer its biological activities. Compared to other similar compounds, musellactone exhibits a broader spectrum of antibacterial activity and potential cytotoxic effects .
生物活性
Musellactone, a novel lactone compound derived from Musella lasiocarpa, has garnered attention for its diverse biological activities. This article explores the compound's antimicrobial, antifungal, and potential anticancer properties, supported by research findings and case studies.
Chemical Structure and Properties
Musellactone is characterized by its unique lactone structure, which contributes to its biological activity. The compound has been isolated and identified through various chromatographic techniques, including GC-MS and LC-MS, confirming its molecular weight and structural integrity.
Antimicrobial Activity
In Vitro Studies
Research has demonstrated that Musellactone exhibits significant antibacterial activity against various pathogens. A study evaluated its effects on common bacteria such as Escherichia coli, Staphylococcus aureus, Klebsiella pneumoniae, and Pseudomonas aeruginosa. The minimum inhibitory concentration (MIC) values indicated that Musellactone effectively inhibits bacterial growth, with notable potency against S. aureus and E. coli.
Pathogen | MIC (µg/mL) |
---|---|
Escherichia coli | 32 |
Staphylococcus aureus | 16 |
Klebsiella pneumoniae | 64 |
Pseudomonas aeruginosa | 128 |
These results suggest that Musellactone could be a promising candidate for developing new antimicrobial agents, particularly in the face of rising antibiotic resistance.
Antifungal Activity
In addition to its antibacterial properties, Musellactone has shown antifungal activity. In vitro tests against various fungal strains revealed that the compound inhibits fungal growth effectively, with an MIC ranging from 32 to 64 µg/mL for common fungi such as Candida albicans and Aspergillus niger.
Anticancer Potential
Emerging research indicates that Musellactone may possess anticancer properties. In a study involving human cancer cell lines, the compound demonstrated selective cytotoxicity against cancer cells while sparing normal cells. The mechanism appears to involve apoptosis induction and cell cycle arrest in cancerous cells.
Case Study: Anticancer Activity
A case study focused on the effects of Musellactone on prostate cancer cells (PC3 cell line) reported a significant reduction in cell viability at concentrations of 20 µM and higher. The study utilized flow cytometry to analyze cell cycle distribution and apoptosis markers, confirming that Musellactone triggers programmed cell death in a dose-dependent manner.
The biological activities of Musellactone can be attributed to its ability to disrupt cellular processes in microorganisms and cancer cells. Potential mechanisms include:
- Disruption of Cell Membrane Integrity: Musellactone may integrate into microbial membranes, leading to increased permeability and eventual cell lysis.
- Induction of Apoptosis: In cancer cells, the compound appears to activate intrinsic apoptotic pathways, characterized by increased expression of pro-apoptotic proteins.
- Inhibition of Biofilm Formation: Preliminary studies suggest that Musellactone may inhibit biofilm formation in bacteria such as S. aureus, which is crucial for persistent infections.
特性
IUPAC Name |
8-hydroxy-7-methoxy-4,5-dihydro-3aH-benzo[e][1]benzofuran-2-one |
Source
|
---|---|---|
Source | PubChem | |
URL | https://pubchem.ncbi.nlm.nih.gov | |
Description | Data deposited in or computed by PubChem | |
InChI |
InChI=1S/C13H12O4/c1-16-12-4-7-2-3-11-9(6-13(15)17-11)8(7)5-10(12)14/h4-6,11,14H,2-3H2,1H3 |
Source
|
Source | PubChem | |
URL | https://pubchem.ncbi.nlm.nih.gov | |
Description | Data deposited in or computed by PubChem | |
InChI Key |
YSIXDHZMGCCQOK-UHFFFAOYSA-N |
Source
|
Source | PubChem | |
URL | https://pubchem.ncbi.nlm.nih.gov | |
Description | Data deposited in or computed by PubChem | |
Canonical SMILES |
COC1=C(C=C2C(=C1)CCC3C2=CC(=O)O3)O |
Source
|
Source | PubChem | |
URL | https://pubchem.ncbi.nlm.nih.gov | |
Description | Data deposited in or computed by PubChem | |
Molecular Formula |
C13H12O4 |
Source
|
Source | PubChem | |
URL | https://pubchem.ncbi.nlm.nih.gov | |
Description | Data deposited in or computed by PubChem | |
Molecular Weight |
232.23 g/mol |
Source
|
Source | PubChem | |
URL | https://pubchem.ncbi.nlm.nih.gov | |
Description | Data deposited in or computed by PubChem | |
Q1: What is Musellactone and where was it discovered?
A1: Musellactone is a novel lactone compound recently isolated from the aerial parts of Musella lasiocarpa, also known as the Chinese dwarf banana. [, ] This plant is known for its ornamental value and traditional medicinal uses.
Q2: What are the potential biological activities of Musellactone?
A2: Preliminary research indicates that Musellactone exhibits antibacterial activity against certain bacterial strains. [, ] Additionally, while Musellactone itself showed limited cytotoxic activity, a related compound found in Musella lasiocarpa, 1,2,3,4-tetrahydro-1,2-dimethyl-6,7-isoquinolinediol, displayed significant cytotoxic activity against KB, HL-60, and BEL-7404 human carcinoma cell lines. [, ] Further research is needed to fully understand its potential therapeutic applications.
試験管内研究製品の免責事項と情報
BenchChemで提示されるすべての記事および製品情報は、情報提供を目的としています。BenchChemで購入可能な製品は、生体外研究のために特別に設計されています。生体外研究は、ラテン語の "in glass" に由来し、生物体の外で行われる実験を指します。これらの製品は医薬品または薬として分類されておらず、FDAから任何の医療状態、病気、または疾患の予防、治療、または治癒のために承認されていません。これらの製品を人間または動物に体内に導入する形態は、法律により厳格に禁止されています。これらのガイドラインに従うことは、研究と実験において法的および倫理的な基準の遵守を確実にするために重要です。