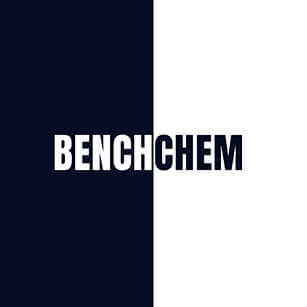
Desmethyl Cyclobenzaprine N-β-D-Glucuronide
- 専門家チームからの見積もりを受け取るには、QUICK INQUIRYをクリックしてください。
- 品質商品を競争力のある価格で提供し、研究に集中できます。
説明
Desmethyl Cyclobenzaprine N-β-D-Glucuronide is a biochemical compound with the molecular formula C25H27NO6 and a molecular weight of 437.48 g/mol . It is a metabolite of cyclobenzaprine, a muscle relaxant commonly used to relieve skeletal muscle spasms and associated pain from acute musculoskeletal conditions . This compound is primarily used in proteomics research and is not intended for diagnostic or therapeutic use .
準備方法
The synthesis of Desmethyl Cyclobenzaprine N-β-D-Glucuronide involves the N-demethylation of cyclobenzaprine followed by glucuronidation. The N-demethylation process is typically catalyzed by cytochrome P450 enzymes, particularly CYP3A4 and CYP1A2 . The glucuronidation step involves the conjugation of the demethylated product with glucuronic acid, facilitated by UDP-glucuronosyltransferase enzymes .
化学反応の分析
Desmethyl Cyclobenzaprine N-β-D-Glucuronide undergoes several types of chemical reactions, including:
Oxidation: This reaction can occur at various positions on the molecule, often facilitated by oxidizing agents such as hydrogen peroxide or potassium permanganate.
Reduction: Reduction reactions are less common but can be achieved using reducing agents like sodium borohydride.
Substitution: Nucleophilic substitution reactions can occur, particularly at the nitrogen atom, using reagents like alkyl halides.
科学的研究の応用
Desmethyl Cyclobenzaprine N-β-D-Glucuronide is primarily used in scientific research, particularly in the fields of:
Chemistry: It serves as a reference standard in analytical chemistry for the quantification of cyclobenzaprine metabolites.
Biology: The compound is used to study metabolic pathways and enzyme kinetics involving glucuronidation.
Medicine: Research involving this compound helps in understanding the pharmacokinetics and pharmacodynamics of cyclobenzaprine and its metabolites.
Industry: It is used in the development of analytical methods for drug testing and forensic toxicology.
作用機序
The mechanism of action of Desmethyl Cyclobenzaprine N-β-D-Glucuronide is closely related to that of its parent compound, cyclobenzaprine. Cyclobenzaprine acts as a 5-HT2 receptor antagonist, relieving muscle spasms through its action on the central nervous system at the brain stem . The glucuronidation of desmethyl cyclobenzaprine enhances its solubility and facilitates its excretion via the kidneys .
類似化合物との比較
Desmethyl Cyclobenzaprine N-β-D-Glucuronide can be compared to other glucuronidated metabolites of tricyclic compounds, such as:
Amitriptyline N-β-D-Glucuronide: Similar in structure and metabolic pathway, but derived from the antidepressant amitriptyline.
Nortriptyline N-β-D-Glucuronide: Another tricyclic antidepressant metabolite with similar glucuronidation.
Clomipramine N-β-D-Glucuronide: Derived from clomipramine, used in the treatment of obsessive-compulsive disorder.
The uniqueness of this compound lies in its specific application in studying the metabolism of cyclobenzaprine and its role in muscle relaxation therapy .
生物活性
Desmethyl cyclobenzaprine N-β-D-glucuronide is a significant metabolite of cyclobenzaprine, a muscle relaxant commonly prescribed for acute musculoskeletal conditions. Understanding the biological activity of this compound is crucial for evaluating its therapeutic efficacy, safety profile, and potential drug interactions.
This compound is primarily formed through the metabolism of cyclobenzaprine, which acts as a 5-HT2 receptor antagonist . This action contributes to its muscle relaxant properties by modulating neurotransmitter levels in the central nervous system (CNS), particularly in the brainstem. Cyclobenzaprine's mechanism involves the inhibition of serotonin and norepinephrine reuptake, which plays a role in pain perception and muscle tone regulation .
Pharmacokinetics
The pharmacokinetics of this compound are influenced by its metabolic pathways. Cyclobenzaprine undergoes extensive liver metabolism via cytochrome P450 enzymes, particularly CYP3A4 and CYP1A2, leading to the formation of desmethyl cyclobenzaprine and subsequent glucuronidation to form its glucuronide conjugate . The elimination of this metabolite occurs primarily through renal excretion.
Clinical Implications
Clinical studies have shown that cyclobenzaprine effectively reduces muscle spasms and associated pain in acute conditions. However, its long-term use is discouraged due to limited evidence supporting its efficacy beyond two weeks and potential side effects . The role of this compound in these outcomes is still under investigation, particularly regarding its contribution to therapeutic effects or adverse reactions.
Case Study Analysis
Recent literature reviews have highlighted various case studies involving cyclobenzaprine and its metabolites. For instance, one study reported adverse events associated with the use of cyclobenzaprine, emphasizing the importance of monitoring patients for potential side effects when using this medication . Another study focused on the pharmacokinetics and bioequivalence evaluation of different formulations of cyclobenzaprine tablets, providing insights into how this compound may influence therapeutic outcomes .
Research Findings Summary Table
特性
CAS番号 |
67200-84-4 |
---|---|
分子式 |
C25H27NO6 |
分子量 |
437.492 |
IUPAC名 |
(2S,3S,4S,5R,6R)-6-[3-(dibenzo[1,2-a:1/',2/'-e][7]annulen-11-ylidene)propyl-methylamino]-3,4,5-trihydroxyoxane-2-carboxylic acid |
InChI |
InChI=1S/C25H27NO6/c1-26(24-22(29)20(27)21(28)23(32-24)25(30)31)14-6-11-19-17-9-4-2-7-15(17)12-13-16-8-3-5-10-18(16)19/h2-5,7-13,20-24,27-29H,6,14H2,1H3,(H,30,31)/t20-,21-,22+,23-,24+/m0/s1 |
InChIキー |
LOSRUFDBUVVKIR-OSFFKXSWSA-N |
SMILES |
CN(CCC=C1C2=CC=CC=C2C=CC3=CC=CC=C31)C4C(C(C(C(O4)C(=O)O)O)O)O |
同義語 |
1-Deoxy-1-[[3-(5H-dibenzo[a,d]cyclohepten-5-ylidene)propyl]methylamino]-β-D-glucopyranuronic Acid; |
製品の起源 |
United States |
試験管内研究製品の免責事項と情報
BenchChemで提示されるすべての記事および製品情報は、情報提供を目的としています。BenchChemで購入可能な製品は、生体外研究のために特別に設計されています。生体外研究は、ラテン語の "in glass" に由来し、生物体の外で行われる実験を指します。これらの製品は医薬品または薬として分類されておらず、FDAから任何の医療状態、病気、または疾患の予防、治療、または治癒のために承認されていません。これらの製品を人間または動物に体内に導入する形態は、法律により厳格に禁止されています。これらのガイドラインに従うことは、研究と実験において法的および倫理的な基準の遵守を確実にするために重要です。