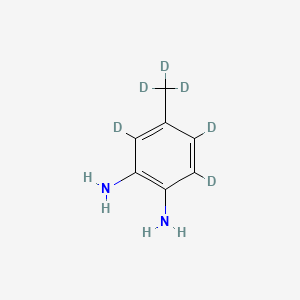
3,4-Diaminotoluene-d6
説明
3,4-Diaminotoluene-d6 is a colorless to brownish purple crystalline solid . It is toxic by ingestion and inhalation and an irritant to skin and eyes . It is soluble in water, alcohol, and ether . It decomposes to emit toxic oxides of nitrogen when heated to high temperature .
Synthesis Analysis
The synthesis method of 3,4-Diaminotoluene involves a hydrogenation reduction reaction performed by taking o-nitro-p-toluidine as a raw material under the action of a catalyst and an alcohol solvent . The weight ratio of the solvent to o-nitro-p-toluidine is (1.5-3):1 . In the hydrogenation reduction reaction, the temperature is 65-85 DEG C and the pressure is 1.0-4.0 MPa .Molecular Structure Analysis
The linear formula of 3,4-Diaminotoluene-d6 is CH3C6H3(NH2)2 . Its CAS Number is 496-72-0 and its molecular weight is 122.17 .Chemical Reactions Analysis
3,4-Diaminotoluene-d6 neutralizes acids in exothermic reactions to form salts plus water . It may be incompatible with isocyanates, halogenated organics, peroxides, phenols (acidic), epoxides, anhydrides, and acid halides . Flammable gaseous hydrogen may be generated in combination with strong reducing agents, such as hydrides .Physical And Chemical Properties Analysis
3,4-Diaminotoluene-d6 is a colorless to brownish purple crystalline solid . It is soluble in water, alcohol, and ether . It decomposes to emit toxic oxides of nitrogen when heated to high temperature .科学的研究の応用
Optoelectronic Applications
3,4-Diaminotoluene-d6 is used in the synthesis of Schiff base ligand, which is then used for complexation with various metals such as Mn, Fe, Co, Ni, Cu, and Zn . These complexes have been studied for their potential in optoelectronic applications . The Zn complex, in particular, shows maximum fluorescence intensity and strong glow when exposed to a UV lamp . This makes it a promising candidate for use in display devices .
Research Use Only (RUO) Applications
3,4-Diaminotoluene-d6 is available for research use only (RUO) applications . This means it is used in scientific research and experiments, but not for diagnostic procedures or therapeutic use .
Electrochemical Sensor Applications
3,4-Diaminotoluene-d6 can be used in the fabrication of electrochemical sensors . For instance, it has been used in the synthesis of a nanocomposite that was coated onto a glassy carbon electrode to create a sensor selective toward 3,4-diaminotoluene .
作用機序
Target of Action
3,4-Diaminotoluene-d6, also known as 3,4-DAT, is an organic compound primarily used as a coreactant or raw material in the production of dyes, corrosion inhibitors, and rubber antioxidants . Its specific chemical properties and versatility make it essential in various industrial applications .
Mode of Action
For instance, it neutralizes acids in exothermic reactions to form salts plus water . It may also be incompatible with certain substances such as isocyanates, halogenated organics, peroxides, phenols (acidic), epoxides, anhydrides, and acid halides .
Biochemical Pathways
It has been observed that this compound exhibits promising capacity in treating aniline and 3,4-diaminotoluene as representative amine organic contaminants . The removal reaction involves multiple diffusion driving forces and micro-mechanisms .
Pharmacokinetics
It is known that 3,4-dat is a solid substance with a boiling point of 155-156 °c/18 mmhg and a melting point of 87-89 °c .
Result of Action
It is known to be toxic by ingestion and inhalation and can cause irritation to the skin and eyes . When heated to high temperatures, it decomposes to emit toxic oxides of nitrogen .
Action Environment
The action, efficacy, and stability of 3,4-DAT can be influenced by various environmental factors. For instance, it is insoluble in water , which could affect its distribution and action in aquatic environments. Furthermore, it can react with strong reducing agents, such as hydrides, to generate flammable gaseous hydrogen .
Safety and Hazards
3,4-Diaminotoluene-d6 is toxic by ingestion and inhalation and an irritant to skin and eyes . It is recommended to store this material in a refrigerator . In case of a spill, all sources of ignition should be removed, and the solid spill material should be dampened with acetone and transferred to a suitable container .
特性
IUPAC Name |
3,4,6-trideuterio-5-(trideuteriomethyl)benzene-1,2-diamine | |
---|---|---|
Source | PubChem | |
URL | https://pubchem.ncbi.nlm.nih.gov | |
Description | Data deposited in or computed by PubChem | |
InChI |
InChI=1S/C7H10N2/c1-5-2-3-6(8)7(9)4-5/h2-4H,8-9H2,1H3/i1D3,2D,3D,4D | |
Source | PubChem | |
URL | https://pubchem.ncbi.nlm.nih.gov | |
Description | Data deposited in or computed by PubChem | |
InChI Key |
DGRGLKZMKWPMOH-RLTMCGQMSA-N | |
Source | PubChem | |
URL | https://pubchem.ncbi.nlm.nih.gov | |
Description | Data deposited in or computed by PubChem | |
Canonical SMILES |
CC1=CC(=C(C=C1)N)N | |
Source | PubChem | |
URL | https://pubchem.ncbi.nlm.nih.gov | |
Description | Data deposited in or computed by PubChem | |
Isomeric SMILES |
[2H]C1=C(C(=C(C(=C1C([2H])([2H])[2H])[2H])N)N)[2H] | |
Source | PubChem | |
URL | https://pubchem.ncbi.nlm.nih.gov | |
Description | Data deposited in or computed by PubChem | |
Molecular Formula |
C7H10N2 | |
Source | PubChem | |
URL | https://pubchem.ncbi.nlm.nih.gov | |
Description | Data deposited in or computed by PubChem | |
Molecular Weight |
128.20 g/mol | |
Source | PubChem | |
URL | https://pubchem.ncbi.nlm.nih.gov | |
Description | Data deposited in or computed by PubChem | |
Retrosynthesis Analysis
AI-Powered Synthesis Planning: Our tool employs the Template_relevance Pistachio, Template_relevance Bkms_metabolic, Template_relevance Pistachio_ringbreaker, Template_relevance Reaxys, Template_relevance Reaxys_biocatalysis model, leveraging a vast database of chemical reactions to predict feasible synthetic routes.
One-Step Synthesis Focus: Specifically designed for one-step synthesis, it provides concise and direct routes for your target compounds, streamlining the synthesis process.
Accurate Predictions: Utilizing the extensive PISTACHIO, BKMS_METABOLIC, PISTACHIO_RINGBREAKER, REAXYS, REAXYS_BIOCATALYSIS database, our tool offers high-accuracy predictions, reflecting the latest in chemical research and data.
Strategy Settings
Precursor scoring | Relevance Heuristic |
---|---|
Min. plausibility | 0.01 |
Model | Template_relevance |
Template Set | Pistachio/Bkms_metabolic/Pistachio_ringbreaker/Reaxys/Reaxys_biocatalysis |
Top-N result to add to graph | 6 |
Feasible Synthetic Routes
試験管内研究製品の免責事項と情報
BenchChemで提示されるすべての記事および製品情報は、情報提供を目的としています。BenchChemで購入可能な製品は、生体外研究のために特別に設計されています。生体外研究は、ラテン語の "in glass" に由来し、生物体の外で行われる実験を指します。これらの製品は医薬品または薬として分類されておらず、FDAから任何の医療状態、病気、または疾患の予防、治療、または治癒のために承認されていません。これらの製品を人間または動物に体内に導入する形態は、法律により厳格に禁止されています。これらのガイドラインに従うことは、研究と実験において法的および倫理的な基準の遵守を確実にするために重要です。