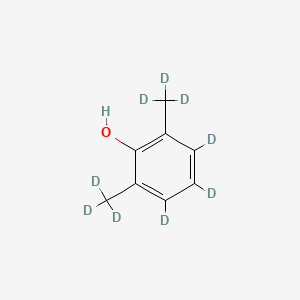
2,6-Dimethylphenol-d9 (Major)
概要
説明
2,6-Dimethylphenol-d9 (Major) is a deuterated form of 2,6-dimethylphenol, also known as 2,6-xylenol. This compound is characterized by the presence of deuterium atoms, which are isotopes of hydrogen, replacing the hydrogen atoms in the molecule. The molecular formula of 2,6-Dimethylphenol-d9 is C8HD9O, and it has a molecular weight of 131.22 . This compound is primarily used in scientific research, particularly in the fields of chemistry and biochemistry, due to its unique isotopic labeling.
準備方法
The synthesis of 2,6-Dimethylphenol-d9 involves the incorporation of deuterium into the 2,6-dimethylphenol molecule. One common method for preparing deuterated compounds is through the use of deuterated reagents or solvents during the synthesis process. For example, deuterated water (D2O) or deuterated acids can be used to introduce deuterium atoms into the target molecule. The specific synthetic routes and reaction conditions for 2,6-Dimethylphenol-d9 can vary, but they generally involve the use of deuterated starting materials and controlled reaction environments to ensure the incorporation of deuterium.
化学反応の分析
2,6-Dimethylphenol-d9 undergoes various chemical reactions similar to its non-deuterated counterpart. These reactions include:
Oxidation: 2,6-Dimethylphenol-d9 can be oxidized to form corresponding quinones or other oxidation products. Common oxidizing agents include potassium permanganate (KMnO4) and chromium trioxide (CrO3).
Reduction: Reduction reactions can convert 2,6-Dimethylphenol-d9 to its corresponding alcohols or other reduced forms. Sodium borohydride (NaBH4) and lithium aluminum hydride (LiAlH4) are typical reducing agents used in these reactions.
Substitution: The phenolic hydroxyl group in 2,6-Dimethylphenol-d9 can undergo substitution reactions, such as alkylation or acylation, to form various derivatives.
科学的研究の応用
2,6-Dimethylphenol-d9 is widely used in scientific research due to its isotopic labeling, which allows for detailed studies of reaction mechanisms and molecular interactions. Some of its applications include:
Chemistry: Used as a tracer in reaction mechanism studies to understand the pathways and intermediates involved in chemical reactions.
Biology: Employed in metabolic studies to trace the incorporation and transformation of phenolic compounds in biological systems.
Medicine: Utilized in pharmacokinetic studies to investigate the absorption, distribution, metabolism, and excretion of drugs containing phenolic moieties.
Industry: Applied in the synthesis of deuterated polymers and materials for specialized applications
作用機序
The mechanism of action of 2,6-Dimethylphenol-d9 is primarily related to its role as a labeled compound in research studies. The deuterium atoms in the molecule provide a distinct isotopic signature that can be detected using various analytical techniques, such as nuclear magnetic resonance (NMR) spectroscopy and mass spectrometry. This allows researchers to track the compound’s behavior and interactions in different environments. The molecular targets and pathways involved depend on the specific application and the nature of the study being conducted .
類似化合物との比較
2,6-Dimethylphenol-d9 is unique due to its deuterium labeling, which distinguishes it from other similar compounds. Some similar compounds include:
2,6-Dimethylphenol: The non-deuterated form of 2,6-Dimethylphenol-d9, commonly used in various chemical and industrial applications.
2,4-Dimethylphenol: Another isomer of dimethylphenol, differing in the position of the methyl groups on the phenol ring.
2,5-Dimethylphenol: Similar to 2,6-Dimethylphenol but with methyl groups at the 2 and 5 positions on the phenol ring.
The deuterium labeling in 2,6-Dimethylphenol-d9 provides unique advantages in research applications, such as enhanced sensitivity in analytical detection and the ability to study reaction mechanisms in greater detail.
特性
IUPAC Name |
3,4,5-trideuterio-2,6-bis(trideuteriomethyl)phenol | |
---|---|---|
Source | PubChem | |
URL | https://pubchem.ncbi.nlm.nih.gov | |
Description | Data deposited in or computed by PubChem | |
InChI |
InChI=1S/C8H10O/c1-6-4-3-5-7(2)8(6)9/h3-5,9H,1-2H3/i1D3,2D3,3D,4D,5D | |
Source | PubChem | |
URL | https://pubchem.ncbi.nlm.nih.gov | |
Description | Data deposited in or computed by PubChem | |
InChI Key |
NXXYKOUNUYWIHA-XVGWXEQOSA-N | |
Source | PubChem | |
URL | https://pubchem.ncbi.nlm.nih.gov | |
Description | Data deposited in or computed by PubChem | |
Canonical SMILES |
CC1=C(C(=CC=C1)C)O | |
Source | PubChem | |
URL | https://pubchem.ncbi.nlm.nih.gov | |
Description | Data deposited in or computed by PubChem | |
Isomeric SMILES |
[2H]C1=C(C(=C(C(=C1[2H])C([2H])([2H])[2H])O)C([2H])([2H])[2H])[2H] | |
Source | PubChem | |
URL | https://pubchem.ncbi.nlm.nih.gov | |
Description | Data deposited in or computed by PubChem | |
Molecular Formula |
C8H10O | |
Source | PubChem | |
URL | https://pubchem.ncbi.nlm.nih.gov | |
Description | Data deposited in or computed by PubChem | |
Molecular Weight |
131.22 g/mol | |
Source | PubChem | |
URL | https://pubchem.ncbi.nlm.nih.gov | |
Description | Data deposited in or computed by PubChem | |
Synthesis routes and methods I
Procedure details
Synthesis routes and methods II
Procedure details
Synthesis routes and methods III
Procedure details
Synthesis routes and methods IV
Procedure details
Synthesis routes and methods V
Procedure details
試験管内研究製品の免責事項と情報
BenchChemで提示されるすべての記事および製品情報は、情報提供を目的としています。BenchChemで購入可能な製品は、生体外研究のために特別に設計されています。生体外研究は、ラテン語の "in glass" に由来し、生物体の外で行われる実験を指します。これらの製品は医薬品または薬として分類されておらず、FDAから任何の医療状態、病気、または疾患の予防、治療、または治癒のために承認されていません。これらの製品を人間または動物に体内に導入する形態は、法律により厳格に禁止されています。これらのガイドラインに従うことは、研究と実験において法的および倫理的な基準の遵守を確実にするために重要です。