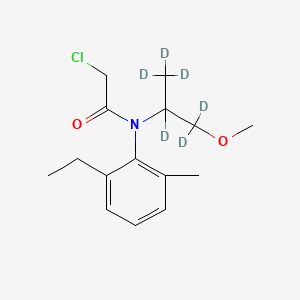
Metolachlor-d6
説明
Metolachlor-d6 is the deuterium labeled Metolachlor . Metolachlor is a pre-emergent selective, chloroacetanilide herbicide used for controlling a variety of annual grass and broadleaf weeds in corn and other crops . It is a chiral herbicide consisting of four stereoisomers .
Synthesis Analysis
Metolachlor is produced from 2-ethyl-6-methylaniline (MEA) via condensation with methoxy acetone. The resulting imine is hydrogenated to give primarily the S-stereoisomeric amine. This secondary amine is acetylated with chloroacetylchloride .Molecular Structure Analysis
The molecular formula of Metolachlor-d6 is C15 2H6 H16 Cl N O2 .Chemical Reactions Analysis
Metolachlor acts by inhibition of elongases and of the geranylgeranyl pyrophosphate (GGPP) cyclases, which are part of the gibberellin pathway . It is used for grass and broadleaf weed control in corn, soybean, peanuts, sorghum, and cotton .Physical And Chemical Properties Analysis
Metolachlor-d6 has a molecular weight of 289.83 .科学的研究の応用
Environmental Science and Pollution Research
A study was conducted on the leaching and degradation of S-Metolachlor in undisturbed soil cores amended with organic wastes . The study aimed at evaluating the balance of these mechanisms under more realistic conditions to clarify the influence of three common organic waste amendments on the fate, in soil, of the widely used herbicide S-Metolachlor . The results showed that S-Metolachlor leaching didn’t exceed 0.1% of the applied dose with or without organic waste amendment .
Soil Remediation
The fungal strain MET-F-1, characterized as Penicillium oxalicum, was isolated from an activated sludge . This strain could degrade 88.6% of 50 mg/L metolachlor coupled with 0.1% glucose plus 0.1% yeast extract within 384 hours under optimal conditions . Field plot experiments using the wheat bran inoculum method were performed and demonstrated good metolachlor-degrading activity of this strain .
Herbicide Resistance
Metolachlor is extensively used and the most persistent chloroacetamide herbicide . In a study, plants from six of the 12 Palmer amaranth accessions that survived a 1 pint/acre rate of Dual Magnum® were analyzed for overexpression of genes associated with resistance to chloroacetamide herbicides .
Groundwater Contamination
Metolachlor and its metabolites have been frequently detected in soils and surface and groundwaters . Concentration of S-Metolachlor and metabolites in the leachates of all treatments greatly exceeded the regulatory limit for groundwater intended for human consumption in Europe .
Microbial Degradation
Microbial degradation is predominantly responsible for the removal of metolachlor from soil and water . Different degradation products, i.e., MOXA, M2H, and MDES, detected by high-performance liquid chromatography-tandem mass spectrometry (HPLC-MS/MS), were produced through hydrolytic and reductive dechlorination by MET-F-1 .
Agricultural Practices
The application of organic waste in agriculture is a common practice fostered by benefits in terms of waste recycling and crop production . However, organic waste amendments potentially affect the fate of pesticide spread on fields to protect the crops from pests and weeds .
作用機序
Target of Action
Metolachlor-d6, like its parent compound Metolachlor, is a part of the chloroacetamide chemical family and belongs to the Weed Science Society of America (WSSA) Group 15 herbicide class . The primary targets of Metolachlor-d6 are the very long chain fatty acids in plants . These fatty acids play a crucial role in the growth and development of seedling shoots .
Mode of Action
Metolachlor-d6 inhibits the formation of very long chain fatty acids, thereby inhibiting seedling shoot growth . This interaction with its targets results in the control of a variety of annual grasses and broadleaf weeds in crops .
Pharmacokinetics
It is known that the compound is used as a pre-emergent herbicide, which suggests that it is applied to the soil and absorbed by the plants from there . The presence of deuterium in Metolachlor-d6 could potentially affect its pharmacokinetic and metabolic profiles .
Result of Action
The result of Metolachlor-d6 action is the effective control of a variety of annual grasses and broadleaf weeds in crops . In terms of its effect on non-target organisms, there is no clear link between S-metolachlor exposure and incidence of cancer in humans .
Action Environment
The action of Metolachlor-d6 can be influenced by environmental factors. For instance, microbial degradation is predominantly responsible for the removal of Metolachlor from soil and water . Furthermore, organic waste amendments can modify the metabolic pathways of S-Metolachlor .
Safety and Hazards
Metolachlor has been detected in ground and surface waters in concentrations ranging from 0.08 to 4.5 parts per billion (ppb) throughout the U.S . It is classified as a Category C pesticide by the United States Environmental Protection Agency (US EPA), which indicates limited evidence of carcinogenicity .
特性
IUPAC Name |
2-chloro-N-(2-ethyl-6-methylphenyl)-N-(1,1,1,2,3,3-hexadeuterio-3-methoxypropan-2-yl)acetamide | |
---|---|---|
Source | PubChem | |
URL | https://pubchem.ncbi.nlm.nih.gov | |
Description | Data deposited in or computed by PubChem | |
InChI |
InChI=1S/C15H22ClNO2/c1-5-13-8-6-7-11(2)15(13)17(14(18)9-16)12(3)10-19-4/h6-8,12H,5,9-10H2,1-4H3/i3D3,10D2,12D | |
Source | PubChem | |
URL | https://pubchem.ncbi.nlm.nih.gov | |
Description | Data deposited in or computed by PubChem | |
InChI Key |
WVQBLGZPHOPPFO-XCPSXBTFSA-N | |
Source | PubChem | |
URL | https://pubchem.ncbi.nlm.nih.gov | |
Description | Data deposited in or computed by PubChem | |
Canonical SMILES |
CCC1=CC=CC(=C1N(C(C)COC)C(=O)CCl)C | |
Source | PubChem | |
URL | https://pubchem.ncbi.nlm.nih.gov | |
Description | Data deposited in or computed by PubChem | |
Isomeric SMILES |
[2H]C([2H])([2H])C([2H])(C([2H])([2H])OC)N(C1=C(C=CC=C1CC)C)C(=O)CCl | |
Source | PubChem | |
URL | https://pubchem.ncbi.nlm.nih.gov | |
Description | Data deposited in or computed by PubChem | |
Molecular Formula |
C15H22ClNO2 | |
Source | PubChem | |
URL | https://pubchem.ncbi.nlm.nih.gov | |
Description | Data deposited in or computed by PubChem | |
DSSTOX Substance ID |
DTXSID401010012 | |
Record name | Metolachlor-d6 | |
Source | EPA DSSTox | |
URL | https://comptox.epa.gov/dashboard/DTXSID401010012 | |
Description | DSSTox provides a high quality public chemistry resource for supporting improved predictive toxicology. | |
Molecular Weight |
289.83 g/mol | |
Source | PubChem | |
URL | https://pubchem.ncbi.nlm.nih.gov | |
Description | Data deposited in or computed by PubChem | |
Product Name |
Metolachlor-d6 | |
CAS RN |
1219803-97-0 | |
Record name | Metolachlor-d6 | |
Source | EPA DSSTox | |
URL | https://comptox.epa.gov/dashboard/DTXSID401010012 | |
Description | DSSTox provides a high quality public chemistry resource for supporting improved predictive toxicology. | |
Q & A
Q1: Why is Metolachlor-d6 used in this study instead of regular Metolachlor?
A1: Metolachlor-d6 serves as an internal standard in this research []. Internal standards are crucial in analytical chemistry, especially when using techniques like mass spectrometry. They are added to samples, calibration standards, and quality control samples at a known concentration.
Q2: How does the use of Metolachlor-d6 improve the reliability of Metolachlor quantification in environmental water samples?
A2: The study demonstrates that matrix effects can significantly impact the accurate determination of Metolachlor in different water types []. By using Metolachlor-d6, the researchers could effectively correct for these matrix effects:
試験管内研究製品の免責事項と情報
BenchChemで提示されるすべての記事および製品情報は、情報提供を目的としています。BenchChemで購入可能な製品は、生体外研究のために特別に設計されています。生体外研究は、ラテン語の "in glass" に由来し、生物体の外で行われる実験を指します。これらの製品は医薬品または薬として分類されておらず、FDAから任何の医療状態、病気、または疾患の予防、治療、または治癒のために承認されていません。これらの製品を人間または動物に体内に導入する形態は、法律により厳格に禁止されています。これらのガイドラインに従うことは、研究と実験において法的および倫理的な基準の遵守を確実にするために重要です。