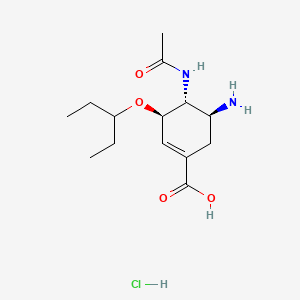
Oseltamivir Acid Hydrochloride
概要
説明
Oseltamivir Acid Hydrochloride is a derivative of Oseltamivir, an antiviral medication primarily used to treat and prevent influenza A and influenza B, the viruses responsible for the flu . Oseltamivir is marketed under the brand name Tamiflu and is known for its role in inhibiting the neuraminidase enzyme, which is crucial for the replication and spread of influenza viruses .
作用機序
- The role of neuraminidase is to cleave sialic acid residues from host cells and budding virions during the viral replication process .
- By interfering with sialic acid cleavage, oseltamivir carboxylate limits the spread of the virus within the host .
- However, the clinical benefit is most significant when administered within 48 hours of symptom onset .
Target of Action
Mode of Action
Biochemical Pathways
Pharmacokinetics
Result of Action
Action Environment
生化学分析
Biochemical Properties
Oseltamivir Carboxylate Hydrochloride exerts its antiviral activity by inhibiting the activity of the viral neuraminidase enzyme found on the surface of the virus . This prevents budding from the host cell, viral replication, and infectivity . It interacts with the neuraminidase enzyme of the Influenza A virus .
Cellular Effects
Oseltamivir Carboxylate Hydrochloride has high bioavailability and penetrates sites of infection at concentrations that are sufficient to inhibit viral replication . It influences cell function by preventing the release of progeny virions from infected host cells, thereby limiting viral load and course of infection .
Molecular Mechanism
Oseltamivir Carboxylate Hydrochloride exerts its effects at the molecular level by binding to and inhibiting the active site of the neuraminidase enzymes . This prevents the release of progeny virions from infected host cells .
Temporal Effects in Laboratory Settings
The stability of Oseltamivir Carboxylate Hydrochloride in plasma was investigated under different conditions . The conversion of the prodrug to the active metabolite was slow and limited in human and rat brain S9 fractions .
Dosage Effects in Animal Models
In animal models, the in vivo efficacy of oseltamivir is lower against viruses that show reduced inhibition by oseltamivir in laboratory testing .
Metabolic Pathways
Oseltamivir Carboxylate Hydrochloride is the active metabolite of the prodrug oseltamivir phosphate, which is rapidly converted by hepatic esterases .
Transport and Distribution
The mean volume of distribution at steady state of Oseltamivir Carboxylate Hydrochloride ranges approximately between 23 and 26 liters in humans, a volume that is roughly equivalent to extracellular body fluid .
Subcellular Localization
The extent of partitioning of Oseltamivir Carboxylate Hydrochloride to the central nervous system is low . Brain-to-plasma exposure ratios were approximately 0.2 for oseltamivir and 0.01 for Oseltamivir Carboxylate Hydrochloride .
準備方法
Synthetic Routes and Reaction Conditions: The synthesis of Oseltamivir Acid Hydrochloride involves multiple steps, starting from shikimic acid, a naturally occurring compound. The process includes the following key steps:
Protection and Functionalization: Shikimic acid undergoes protection of the hydroxyl groups and functionalization to introduce the necessary functional groups.
Cyclization and Amination: The protected intermediate is cyclized and then aminated to introduce the amino group.
Deprotection and Esterification: The protected groups are removed, and the compound is esterified to form Oseltamivir.
Hydrolysis and Salt Formation: Oseltamivir is hydrolyzed to form Oseltamivir Acid, which is then converted to its hydrochloride salt.
Industrial Production Methods: Industrial production of this compound follows a similar synthetic route but is optimized for large-scale production. This involves:
Efficient Catalysts: Use of efficient catalysts to speed up the reactions.
Optimized Reaction Conditions: Control of temperature, pressure, and pH to maximize yield and purity.
Purification Techniques: Advanced purification techniques such as crystallization and chromatography to ensure high purity of the final product.
化学反応の分析
Types of Reactions: Oseltamivir Acid Hydrochloride undergoes various chemical reactions, including:
Hydrolysis: Conversion of Oseltamivir to Oseltamivir Acid.
Esterification: Formation of esters from Oseltamivir Acid.
Salt Formation: Reaction with hydrochloric acid to form this compound.
Common Reagents and Conditions:
Hydrolysis: Typically performed using water or aqueous acids.
Esterification: Involves alcohols and acid catalysts.
Salt Formation: Uses hydrochloric acid under controlled conditions.
Major Products:
Oseltamivir Acid: The primary product of hydrolysis.
This compound: The final product after salt formation.
科学的研究の応用
Oseltamivir Acid Hydrochloride has a wide range of applications in scientific research:
Chemistry: Used as a reference standard in analytical chemistry for the development of analytical methods.
Biology: Studied for its interactions with viral proteins and its inhibitory effects on viral replication.
Medicine: Extensively researched for its efficacy in treating and preventing influenza.
Industry: Used in the pharmaceutical industry for the production of antiviral medications.
類似化合物との比較
Zanamivir: Another neuraminidase inhibitor used to treat influenza.
Peramivir: An intravenous neuraminidase inhibitor for treating influenza.
Laninamivir: A long-acting neuraminidase inhibitor used in some countries for influenza treatment.
Comparison:
Oseltamivir vs. Zanamivir: Oseltamivir is administered orally, while Zanamivir is inhaled.
Oseltamivir vs. Peramivir: Peramivir is administered intravenously and is used in more severe cases of influenza.
Oseltamivir vs. Laninamivir: Laninamivir has a longer duration of action, requiring fewer doses.
Oseltamivir Acid Hydrochloride stands out due to its oral bioavailability and widespread use in treating and preventing influenza, making it a crucial component in antiviral therapy .
特性
IUPAC Name |
(3R,4R,5S)-4-acetamido-5-amino-3-pentan-3-yloxycyclohexene-1-carboxylic acid;hydrochloride | |
---|---|---|
Source | PubChem | |
URL | https://pubchem.ncbi.nlm.nih.gov | |
Description | Data deposited in or computed by PubChem | |
InChI |
InChI=1S/C14H24N2O4.ClH/c1-4-10(5-2)20-12-7-9(14(18)19)6-11(15)13(12)16-8(3)17;/h7,10-13H,4-6,15H2,1-3H3,(H,16,17)(H,18,19);1H/t11-,12+,13+;/m0./s1 | |
Source | PubChem | |
URL | https://pubchem.ncbi.nlm.nih.gov | |
Description | Data deposited in or computed by PubChem | |
InChI Key |
OTBMMOBYSNFNOE-LUHWTZLKSA-N | |
Source | PubChem | |
URL | https://pubchem.ncbi.nlm.nih.gov | |
Description | Data deposited in or computed by PubChem | |
Canonical SMILES |
CCC(CC)OC1C=C(CC(C1NC(=O)C)N)C(=O)O.Cl | |
Source | PubChem | |
URL | https://pubchem.ncbi.nlm.nih.gov | |
Description | Data deposited in or computed by PubChem | |
Isomeric SMILES |
CCC(CC)O[C@@H]1C=C(C[C@@H]([C@H]1NC(=O)C)N)C(=O)O.Cl | |
Source | PubChem | |
URL | https://pubchem.ncbi.nlm.nih.gov | |
Description | Data deposited in or computed by PubChem | |
Molecular Formula |
C14H25ClN2O4 | |
Source | PubChem | |
URL | https://pubchem.ncbi.nlm.nih.gov | |
Description | Data deposited in or computed by PubChem | |
Molecular Weight |
320.81 g/mol | |
Source | PubChem | |
URL | https://pubchem.ncbi.nlm.nih.gov | |
Description | Data deposited in or computed by PubChem | |
Retrosynthesis Analysis
AI-Powered Synthesis Planning: Our tool employs the Template_relevance Pistachio, Template_relevance Bkms_metabolic, Template_relevance Pistachio_ringbreaker, Template_relevance Reaxys, Template_relevance Reaxys_biocatalysis model, leveraging a vast database of chemical reactions to predict feasible synthetic routes.
One-Step Synthesis Focus: Specifically designed for one-step synthesis, it provides concise and direct routes for your target compounds, streamlining the synthesis process.
Accurate Predictions: Utilizing the extensive PISTACHIO, BKMS_METABOLIC, PISTACHIO_RINGBREAKER, REAXYS, REAXYS_BIOCATALYSIS database, our tool offers high-accuracy predictions, reflecting the latest in chemical research and data.
Strategy Settings
Precursor scoring | Relevance Heuristic |
---|---|
Min. plausibility | 0.01 |
Model | Template_relevance |
Template Set | Pistachio/Bkms_metabolic/Pistachio_ringbreaker/Reaxys/Reaxys_biocatalysis |
Top-N result to add to graph | 6 |
Feasible Synthetic Routes
試験管内研究製品の免責事項と情報
BenchChemで提示されるすべての記事および製品情報は、情報提供を目的としています。BenchChemで購入可能な製品は、生体外研究のために特別に設計されています。生体外研究は、ラテン語の "in glass" に由来し、生物体の外で行われる実験を指します。これらの製品は医薬品または薬として分類されておらず、FDAから任何の医療状態、病気、または疾患の予防、治療、または治癒のために承認されていません。これらの製品を人間または動物に体内に導入する形態は、法律により厳格に禁止されています。これらのガイドラインに従うことは、研究と実験において法的および倫理的な基準の遵守を確実にするために重要です。