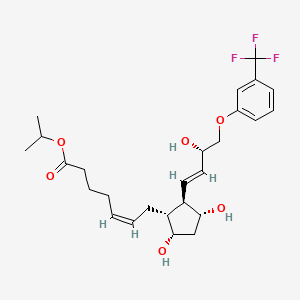
15-epi Travoprost
概要
説明
15-epi Travoprost is a high-quality, certified reference material . It is a synthetic prostaglandin analog, more specifically, an analog of prostaglandin F2α . It is used in the treatment of elevated intraocular pressure due to open-angle glaucoma or ocular hypertension . It works by increasing the outflow of aqueous fluid from the eyes .
Synthesis Analysis
The synthesis of Travoprost involves several steps, including stereoselective reduction, lactone group reduction, removal of the p-phenylbenzoyl protecting group, and transformation of the resulting triol by Wittig reaction into the acid, which is then esterified .Molecular Structure Analysis
The molecular formula of 15-epi Travoprost is C26H35F3O6 . Its molecular weight is 500.55 .Chemical Reactions Analysis
The chemical reactions involved in the synthesis of Travoprost include stereoselective reduction, lactone group reduction, removal of the p-phenylbenzoyl protecting group, and transformation of the resulting triol by Wittig reaction into the acid, which is then esterified .Physical And Chemical Properties Analysis
The physical and chemical properties of 15-epi Travoprost include its molecular formula C26H35F3O6 and molecular weight 500.55 .科学的研究の応用
Treatment of Open-Angle Glaucoma or Ocular Hypertension
Travoprost has been used in the treatment of open-angle glaucoma (OAG) or ocular hypertension (OHT). In a study, the efficacy and safety of the travoprost intracameral SE-implant (slow-eluting implant, the intended commercial product) and FE-implant (fast-eluting implant, included primarily for masking purposes) were evaluated compared to twice-daily (BID) timolol ophthalmic solution, 0.5% in patients with OAG or OHT .
Reduction of Intraocular Pressure
Travoprost has been shown to be effective in reducing intraocular pressure (IOP). In a study, the travoprost intraocular implant was found to be non-inferior to timolol eye drops in IOP lowering over the first 3 months, and was also non-inferior to timolol at months 6, 9, and 12 .
Long-Term Effectiveness in Glaucoma Treatment
A multicenter retrospective cohort study compared the effectiveness and safety of long-term tafluprost, travoprost, or latanoprost in patients with primary open-angle glaucoma (POAG) or normal-tension glaucoma (NTG). The study found that these medications were effective in the long-term treatment of these conditions .
Reduction of Topical Glaucoma Medications
In a study, it was found that a significantly greater proportion of patients in the SE- and FE-implant groups (83.5% and 78.7%, respectively) compared to the timolol group (23.9%) were on fewer topical glaucoma medications at month 12 compared to screening .
Safety in Long-Term Use
The safety of long-term use of travoprost has been studied. In a study, the travoprost intraocular implant was found to be safe for use over a period of 12 months .
Use in Multicenter Clinical Trials
Travoprost has been used in multicenter, randomized, double-masked, sham-controlled, noninferiority trials to evaluate its safety and intraocular pressure (IOP)-lowering efficacy .
作用機序
Mode of Action
Upon administration, 15-epi Travoprost is absorbed through the cornea and is hydrolyzed to its active metabolite, travoprost free acid . As a full agonist and highly selective at the prostanoid receptor, it demonstrates a higher efficacy in reducing intraocular pressure and a reduced risk for developing off-target side effects .
Biochemical Pathways
This is likely achieved through increased outflow of aqueous humor via the trabecular meshwork, the primary route of outflow for the aqueous humor .
Pharmacokinetics
15-epi Travoprost is well-absorbed through the cornea. After absorption, it is hydrolyzed to its active form, travoprost free acid . Peak plasma concentrations of travoprost free acid occur within 30 minutes . The elimination half-life is approximately 45 minutes , indicating that the compound is rapidly metabolized and eliminated.
Result of Action
The primary result of 15-epi Travoprost action is a reduction in intraocular pressure. This is achieved through its agonistic action on the FP prostanoid receptor, leading to increased outflow of aqueous humor via the trabecular meshwork . This reduction in intraocular pressure helps in the management of conditions like open-angle glaucoma and ocular hypertension .
Safety and Hazards
Travoprost may cause serious eye irritation and may cause respiratory irritation . It is recommended to avoid dust formation, avoid breathing mist, gas or vapors, avoid contacting with skin and eye, use personal protective equipment, wear chemical impermeable gloves, ensure adequate ventilation, remove all sources of ignition, evacuate personnel to safe areas, and keep people away from and upwind of spill/leak .
将来の方向性
特性
IUPAC Name |
propan-2-yl (Z)-7-[(1R,2R,3R,5S)-3,5-dihydroxy-2-[(E,3S)-3-hydroxy-4-[3-(trifluoromethyl)phenoxy]but-1-enyl]cyclopentyl]hept-5-enoate | |
---|---|---|
Source | PubChem | |
URL | https://pubchem.ncbi.nlm.nih.gov | |
Description | Data deposited in or computed by PubChem | |
InChI |
InChI=1S/C26H35F3O6/c1-17(2)35-25(33)11-6-4-3-5-10-21-22(24(32)15-23(21)31)13-12-19(30)16-34-20-9-7-8-18(14-20)26(27,28)29/h3,5,7-9,12-14,17,19,21-24,30-32H,4,6,10-11,15-16H2,1-2H3/b5-3-,13-12+/t19-,21+,22+,23-,24+/m0/s1 | |
Source | PubChem | |
URL | https://pubchem.ncbi.nlm.nih.gov | |
Description | Data deposited in or computed by PubChem | |
InChI Key |
MKPLKVHSHYCHOC-AURHPGDUSA-N | |
Source | PubChem | |
URL | https://pubchem.ncbi.nlm.nih.gov | |
Description | Data deposited in or computed by PubChem | |
Canonical SMILES |
CC(C)OC(=O)CCCC=CCC1C(CC(C1C=CC(COC2=CC=CC(=C2)C(F)(F)F)O)O)O | |
Source | PubChem | |
URL | https://pubchem.ncbi.nlm.nih.gov | |
Description | Data deposited in or computed by PubChem | |
Isomeric SMILES |
CC(C)OC(=O)CCC/C=C\C[C@H]1[C@H](C[C@H]([C@@H]1/C=C/[C@@H](COC2=CC=CC(=C2)C(F)(F)F)O)O)O | |
Source | PubChem | |
URL | https://pubchem.ncbi.nlm.nih.gov | |
Description | Data deposited in or computed by PubChem | |
Molecular Formula |
C26H35F3O6 | |
Source | PubChem | |
URL | https://pubchem.ncbi.nlm.nih.gov | |
Description | Data deposited in or computed by PubChem | |
Molecular Weight |
500.5 g/mol | |
Source | PubChem | |
URL | https://pubchem.ncbi.nlm.nih.gov | |
Description | Data deposited in or computed by PubChem | |
CAS RN |
1420791-14-5 | |
Record name | 15-Epi travoprost | |
Source | ChemIDplus | |
URL | https://pubchem.ncbi.nlm.nih.gov/substance/?source=chemidplus&sourceid=1420791145 | |
Description | ChemIDplus is a free, web search system that provides access to the structure and nomenclature authority files used for the identification of chemical substances cited in National Library of Medicine (NLM) databases, including the TOXNET system. | |
Record name | (+)-(5Z,9α,1α,13E,15S)-trihydroxy-16-(3-(trifluoromethyl)phenoxy)-17,18,19,20-tetranor-prosta-5,13-dien-1-oic acid, isopropyl ester | |
Source | European Chemicals Agency (ECHA) | |
URL | https://echa.europa.eu/information-on-chemicals | |
Description | The European Chemicals Agency (ECHA) is an agency of the European Union which is the driving force among regulatory authorities in implementing the EU's groundbreaking chemicals legislation for the benefit of human health and the environment as well as for innovation and competitiveness. | |
Explanation | Use of the information, documents and data from the ECHA website is subject to the terms and conditions of this Legal Notice, and subject to other binding limitations provided for under applicable law, the information, documents and data made available on the ECHA website may be reproduced, distributed and/or used, totally or in part, for non-commercial purposes provided that ECHA is acknowledged as the source: "Source: European Chemicals Agency, http://echa.europa.eu/". Such acknowledgement must be included in each copy of the material. ECHA permits and encourages organisations and individuals to create links to the ECHA website under the following cumulative conditions: Links can only be made to webpages that provide a link to the Legal Notice page. | |
Record name | 15-EPI TRAVOPROST | |
Source | FDA Global Substance Registration System (GSRS) | |
URL | https://gsrs.ncats.nih.gov/ginas/app/beta/substances/NDD23J941F | |
Description | The FDA Global Substance Registration System (GSRS) enables the efficient and accurate exchange of information on what substances are in regulated products. Instead of relying on names, which vary across regulatory domains, countries, and regions, the GSRS knowledge base makes it possible for substances to be defined by standardized, scientific descriptions. | |
Explanation | Unless otherwise noted, the contents of the FDA website (www.fda.gov), both text and graphics, are not copyrighted. They are in the public domain and may be republished, reprinted and otherwise used freely by anyone without the need to obtain permission from FDA. Credit to the U.S. Food and Drug Administration as the source is appreciated but not required. | |
Retrosynthesis Analysis
AI-Powered Synthesis Planning: Our tool employs the Template_relevance Pistachio, Template_relevance Bkms_metabolic, Template_relevance Pistachio_ringbreaker, Template_relevance Reaxys, Template_relevance Reaxys_biocatalysis model, leveraging a vast database of chemical reactions to predict feasible synthetic routes.
One-Step Synthesis Focus: Specifically designed for one-step synthesis, it provides concise and direct routes for your target compounds, streamlining the synthesis process.
Accurate Predictions: Utilizing the extensive PISTACHIO, BKMS_METABOLIC, PISTACHIO_RINGBREAKER, REAXYS, REAXYS_BIOCATALYSIS database, our tool offers high-accuracy predictions, reflecting the latest in chemical research and data.
Strategy Settings
Precursor scoring | Relevance Heuristic |
---|---|
Min. plausibility | 0.01 |
Model | Template_relevance |
Template Set | Pistachio/Bkms_metabolic/Pistachio_ringbreaker/Reaxys/Reaxys_biocatalysis |
Top-N result to add to graph | 6 |
Feasible Synthetic Routes
試験管内研究製品の免責事項と情報
BenchChemで提示されるすべての記事および製品情報は、情報提供を目的としています。BenchChemで購入可能な製品は、生体外研究のために特別に設計されています。生体外研究は、ラテン語の "in glass" に由来し、生物体の外で行われる実験を指します。これらの製品は医薬品または薬として分類されておらず、FDAから任何の医療状態、病気、または疾患の予防、治療、または治癒のために承認されていません。これらの製品を人間または動物に体内に導入する形態は、法律により厳格に禁止されています。これらのガイドラインに従うことは、研究と実験において法的および倫理的な基準の遵守を確実にするために重要です。