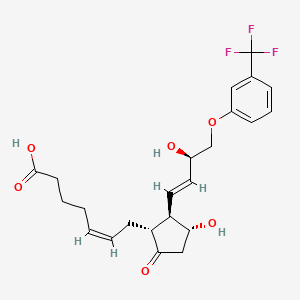
9-ケトフルプロステノール
- 専門家チームからの見積もりを受け取るには、QUICK INQUIRYをクリックしてください。
- 品質商品を競争力のある価格で提供し、研究に集中できます。
説明
9-ケトフルプロステノールは、プロスタグランジンE2(PGE2)の強力なアナログであり、半減期と効力を高めることを目的とした構造修飾が施されています。これは、主にFP受容体と相互作用する、プロスタグランジンF2α(PGF2α)のよく研究されているアナログであるフルプロステノールに由来します。 フルプロステノールの炭素9位での酸化により、9-ケトフルプロステノールが生成され、これはEP受容体に対する高い親和性を示し、PGE2アゴニストとして機能する可能性があります .
科学的研究の応用
9-Keto Fluprostenol has a wide range of applications in scientific research:
Chemistry: Used as a reference compound in the study of prostaglandin analogs and their chemical properties.
Biology: Investigated for its interactions with EP receptors and its role as a PGE2 agonist.
Medicine: Potential therapeutic applications in the treatment of conditions related to prostaglandin pathways, such as glaucoma and other inflammatory diseases.
Industry: Utilized in the development of new pharmaceuticals and as a tool in drug discovery .
準備方法
合成経路と反応条件: 9-ケトフルプロステノールの合成には、フルプロステノールの炭素9位での酸化が伴います。 このプロセスは、制御された条件下で様々な酸化剤を用いて達成することができ、分子中の他の官能基に影響を与えることなくケト基を選択的に生成します .
工業生産方法: 9-ケトフルプロステノールの具体的な工業生産方法は、広く文書化されていませんが、一般的なアプローチは、収量と純度を最大化するように最適化された条件下で、大規模酸化反応を行うことを含みます。 このプロセスには、クロマトグラフィーや結晶化による精製などの工程が含まれ、最終生成物を高純度で得ることが可能になります .
反応の種類:
酸化: 9-ケトフルプロステノールの合成に関与する主要な反応は、フルプロステノールの炭素9位での酸化です。
還元: 潜在的な還元反応は、ケト基をヒドロキシル基に戻すことができますが、これはあまり追求されていません。
一般的な試薬と条件:
酸化剤: 使用される一般的な酸化剤には、三酸化クロム、過マンガン酸カリウム、その他の選択的な酸化剤などがあります。
主要な生成物:
- 酸化反応の主要な生成物は、9-ケトフルプロステノールそのものです。
- その他の潜在的な生成物には、分子のさらなる官能化によって形成された様々な誘導体などがあります .
4. 科学研究への応用
9-ケトフルプロステノールは、科学研究において幅広い用途があります。
化学: プロスタグランジンアナログとその化学的性質の研究における基準化合物として使用されます。
生物学: EP受容体との相互作用と、PGE2アゴニストとしての役割について調査されています。
医学: 緑内障やその他の炎症性疾患など、プロスタグランジン経路に関連する状態の治療における潜在的な治療的用途があります。
作用機序
9-ケトフルプロステノールは、主にEP受容体に対する高い親和性を介して効果を発揮し、PGE2アゴニストとして機能します。分子標的は、炎症、血管拡張、平滑筋収縮など、多くの生理学的プロセスに関与する様々なEP受容体サブタイプです。 これらの受容体の活性化により、化合物の生物学的効果を媒介する下流シグナル伝達経路が誘導されます .
類似化合物:
フルプロステノール: 9-ケトフルプロステノールが誘導された親化合物。主にFP受容体と相互作用します。
プロスタグランジンE2(PGE2): 類似の生物学的活性を持つ天然のプロスタグランジンですが、半減期が短いです。
トラボプロスト: 緑内障の治療に使用されるもう1つのプロスタグランジンアナログであり、代謝されて活性代謝物を生成します .
独自性: 9-ケトフルプロステノールは、他のプロスタグランジンアナログと比較して、半減期と効力を高める構造修飾が施されている点でユニークです。 EP受容体に対する高い親和性と、PGE2アゴニストとしての可能性は、研究および治療的用途に適した化合物となっています .
類似化合物との比較
Fluprostenol: The parent compound from which 9-Keto Fluprostenol is derived. It primarily interacts with FP receptors.
Prostaglandin E2 (PGE2): A naturally occurring prostaglandin with similar biological activities but a shorter half-life.
Travoprost: Another prostaglandin analog used in the treatment of glaucoma, which undergoes metabolism to form active metabolites .
Uniqueness: 9-Keto Fluprostenol is unique due to its structural modifications that enhance its half-life and potency compared to other prostaglandin analogs. Its high affinity for EP receptors and potential as a PGE2 agonist make it a valuable compound for research and therapeutic applications .
特性
IUPAC Name |
(Z)-7-[(1R,2R,3R)-3-hydroxy-2-[(E,3R)-3-hydroxy-4-[3-(trifluoromethyl)phenoxy]but-1-enyl]-5-oxocyclopentyl]hept-5-enoic acid |
Source
|
---|---|---|
Source | PubChem | |
URL | https://pubchem.ncbi.nlm.nih.gov | |
Description | Data deposited in or computed by PubChem | |
InChI |
InChI=1S/C23H27F3O6/c24-23(25,26)15-6-5-7-17(12-15)32-14-16(27)10-11-19-18(20(28)13-21(19)29)8-3-1-2-4-9-22(30)31/h1,3,5-7,10-12,16,18-19,21,27,29H,2,4,8-9,13-14H2,(H,30,31)/b3-1-,11-10+/t16-,18-,19-,21-/m1/s1 |
Source
|
Source | PubChem | |
URL | https://pubchem.ncbi.nlm.nih.gov | |
Description | Data deposited in or computed by PubChem | |
InChI Key |
WWMXHYUXIKWXSR-AAHOZRAYSA-N |
Source
|
Source | PubChem | |
URL | https://pubchem.ncbi.nlm.nih.gov | |
Description | Data deposited in or computed by PubChem | |
Canonical SMILES |
C1C(C(C(C1=O)CC=CCCCC(=O)O)C=CC(COC2=CC=CC(=C2)C(F)(F)F)O)O |
Source
|
Source | PubChem | |
URL | https://pubchem.ncbi.nlm.nih.gov | |
Description | Data deposited in or computed by PubChem | |
Isomeric SMILES |
C1[C@H]([C@@H]([C@H](C1=O)C/C=C\CCCC(=O)O)/C=C/[C@H](COC2=CC=CC(=C2)C(F)(F)F)O)O |
Source
|
Source | PubChem | |
URL | https://pubchem.ncbi.nlm.nih.gov | |
Description | Data deposited in or computed by PubChem | |
Molecular Formula |
C23H27F3O6 |
Source
|
Source | PubChem | |
URL | https://pubchem.ncbi.nlm.nih.gov | |
Description | Data deposited in or computed by PubChem | |
Molecular Weight |
456.5 g/mol |
Source
|
Source | PubChem | |
URL | https://pubchem.ncbi.nlm.nih.gov | |
Description | Data deposited in or computed by PubChem | |
試験管内研究製品の免責事項と情報
BenchChemで提示されるすべての記事および製品情報は、情報提供を目的としています。BenchChemで購入可能な製品は、生体外研究のために特別に設計されています。生体外研究は、ラテン語の "in glass" に由来し、生物体の外で行われる実験を指します。これらの製品は医薬品または薬として分類されておらず、FDAから任何の医療状態、病気、または疾患の予防、治療、または治癒のために承認されていません。これらの製品を人間または動物に体内に導入する形態は、法律により厳格に禁止されています。これらのガイドラインに従うことは、研究と実験において法的および倫理的な基準の遵守を確実にするために重要です。