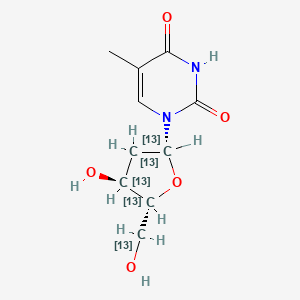
Thymidine-1',2',3',4',5'-13C5
説明
Thymidine-1',2',3',4',5'-13C5 (CAS: 156968-81-9) is a stable isotope-labeled nucleoside where all five carbon atoms in the deoxyribose sugar moiety are replaced with carbon-13 (13C). This compound serves as an internal standard in liquid chromatography–tandem mass spectrometry (LC–MS/MS) for quantifying thymidine in biological matrices such as plasma and urine . Its isotopic purity (>95% by HPLC) and stability under storage conditions (+4°C) make it indispensable for pharmacokinetic and metabolic studies .
準備方法
Chemical Synthesis of the 13C-Labeled Deoxyribose Moiety
The deoxyribose sugar in thymidine-13C5 requires site-specific 13C enrichment. Synthetic routes typically begin with 13C-labeled precursors to construct the 2-deoxyribose structure.
Starting Materials and Isotopic Enrichment
The five-carbon backbone of deoxyribose is synthesized using 13C-enriched glucose or ribose. For example, 13C5-ribose undergoes selective reduction at the 2'-position via the Heyns rearrangement to yield 2-deoxyribonolactone, which is subsequently reduced to 2-deoxyribose . Alternative methods involve:
-
Kost-Sweet reaction : Condensation of 13C-labeled glyceraldehyde with dihydroxyacetone phosphate to form ribose-5-phosphate, followed by enzymatic deoxygenation .
-
Chemical reduction : Treatment of 13C5-ribose derivatives with sodium borohydride (NaBH4) or catalytic hydrogenation to remove the 2'-hydroxyl group .
Protection and Activation
To prevent unwanted side reactions during glycosylation, hydroxyl groups on the 13C-labeled deoxyribose are protected:
-
5'-Hydroxyl : Protected with acid-labile groups like dimethoxytrityl (DMT) .
-
3'-Hydroxyl : Converted to a leaving group (e.g., tosylate or mesylate) for subsequent nucleophilic substitution .
Glycosylation with Thymine
Coupling the 13C-labeled deoxyribose to thymine involves Vorbrüggen glycosylation , a widely used method for nucleoside synthesis.
Reaction Conditions
-
Sugar activation : The protected deoxyribose is silylated using hexamethyldisilazane (HMDS) and trimethylsilyl triflate (TMSOTf) .
-
Nucleobase coupling : Silylated thymine is reacted with the activated sugar under anhydrous conditions, typically in acetonitrile or dichloromethane .
-
Catalysts : Lewis acids like tin(IV) chloride (SnCl4) or boron trifluoride etherate (BF3·OEt2) facilitate β-anomer formation, critical for biological activity .
Stereochemical Control
The reaction’s stereochemical outcome depends on:
-
Protecting groups : Bulky groups at the 5'-position favor β-configuration by hindering α-face attack .
-
Temperature : Lower temperatures (−20°C to 0°C) improve β-selectivity .
Deprotection and Purification
Post-glycosylation, protective groups are removed, and the product is isolated.
Deprotection Steps
-
DMT removal : Treatment with dilute acids (e.g., 2% trifluoroacetic acid in dichloromethane) cleaves the 5'-DMT group .
-
Hydroxyl deprotection : Alkaline conditions (e.g., ammonium hydroxide) hydrolyze remaining esters or carbonates .
Chromatographic Purification
-
Ion-exchange chromatography : Separates unreacted thymine and sugar intermediates .
-
Reverse-phase HPLC : Uses C18 columns with water/acetonitrile gradients to isolate thymidine-13C5 .
Analytical Characterization
Mass Spectrometry
High-resolution mass spectrometry (HRMS) confirms isotopic enrichment and molecular integrity:
-
Observed m/z : 247.19 (calculated for C10H14N2O5 with five 13C atoms) .
-
Isotopic purity : ≥98% 13C5 verified by isotopic abundance analysis .
Nuclear Magnetic Resonance (NMR)
13C NMR spectra validate site-specific labeling:
Yield Optimization and Challenges
Key Factors Affecting Yield
-
Glycosylation efficiency : 60–75% yield under optimized conditions .
-
Isotopic precursor cost : 13C-enriched starting materials account for >80% of total synthesis costs .
Common Pitfalls
-
Anomeric mixture formation : Poor β-selectivity reduces usable product .
-
Isotopic dilution : Trace unlabeled contaminants necessitate rigorous purification .
Comparative Analysis of Synthetic Routes
Method | Advantages | Disadvantages | Yield (%) |
---|---|---|---|
Vorbrüggen glycosylation | High β-selectivity, scalable | Requires anhydrous conditions | 70 |
Enzymatic synthesis | Mild conditions, no protecting groups | Low isotopic incorporation efficiency | 30 |
Solid-phase synthesis | Amenable to automation | High reagent costs | 50 |
Industrial and Research Applications
This compound is indispensable for:
化学反応の分析
Types of Reactions
Thymidine-1’,2’,3’,4’,5’-13C5 undergoes various chemical reactions, including:
Oxidation: The hydroxyl groups in the thymidine molecule can be oxidized to form ketones or aldehydes.
Reduction: The carbonyl groups can be reduced to hydroxyl groups.
Substitution: The nucleoside can undergo nucleophilic substitution reactions, where the hydroxyl groups are replaced by other functional groups.
Common Reagents and Conditions
Common reagents used in these reactions include oxidizing agents like potassium permanganate, reducing agents like sodium borohydride, and nucleophiles like halides. The reactions are typically carried out under controlled conditions to ensure the selective modification of the thymidine molecule.
Major Products
The major products formed from these reactions depend on the specific reagents and conditions used. For example, oxidation of the hydroxyl groups can yield thymidine ketones, while reduction can produce thymidine alcohols.
科学的研究の応用
Thymidine-1’,2’,3’,4’,5’-13C5 has a wide range of applications in scientific research:
Chemistry: Used as a tracer in nuclear magnetic resonance (NMR) spectroscopy to study the structure and dynamics of nucleic acids.
Biology: Employed in metabolic labeling experiments to track DNA synthesis and cell proliferation.
Medicine: Utilized in diagnostic imaging techniques to monitor the distribution and metabolism of thymidine in the body.
Industry: Applied in the development of new pharmaceuticals and biotechnological products
作用機序
Thymidine-1’,2’,3’,4’,5’-13C5 exerts its effects by incorporating into DNA during the synthesis phase of the cell cycle. The labeled thymidine is taken up by cells and incorporated into newly synthesized DNA strands, allowing researchers to track DNA replication and cell division. The molecular targets include thymidine kinase and DNA polymerase, which are involved in the phosphorylation and incorporation of thymidine into DNA .
類似化合物との比較
Structural and Isotopic Labeling Differences
Thymidine-13C5 is compared with other 13C-labeled nucleosides and analogs based on isotopic labeling patterns and structural features (Table 1).
Table 1: Isotopic Labeling and Structural Features
*Calculated based on unlabeled molecular weight + 5×13C contribution.
†Estimated from synthesis protocols .
Key Observations :
- Thymidine-13C5 and 2’-deoxyuridine-13C5 share identical 13C labeling positions but differ in their pyrimidine bases (thymine vs. uracil), affecting their roles in DNA/RNA studies .
- Cytidine-13C5 has a cytosine base and is used in RNA research, whereas thymidine-13C5 is DNA-specific .
- [13C5]-β-dG, a deoxyguanosine analog, incorporates 13C into both sugar and base, enabling DNA replication studies .
Analytical Performance in LC–MS/MS
Retention times and assay performance parameters highlight differences in chromatographic behavior (Table 2).
Table 2: LC–MS/MS Performance Comparison
Compound | Retention Time (minutes) | Carry-Over (%) | Column Used |
---|---|---|---|
Thymidine-13C5 | 2.0 | <2 | Hypercarb™ |
2’-Deoxyuridine-13C5 | 1.2 | 0 | Hypercarb™ |
Unlabeled Thymidine | 2.0 | N/A | Hypercarb™ |
Unlabeled 2’-Deoxyuridine | 1.2 | N/A | Hypercarb™ |
Data from demonstrates that thymidine-13C5 and its unlabeled form co-elute, ensuring accurate quantification. However, thymidine-13C5 exhibits minimal carry-over (<2%), while 2’-deoxyuridine-13C5 shows none, reflecting differences in column interaction .
生物活性
Thymidine-1',2',3',4',5'-13C5 is a stable isotope-labeled form of thymidine, a nucleoside essential for DNA synthesis. This compound has garnered attention in biological research due to its potential applications in metabolic studies, cancer research, and as a tracer in various biochemical pathways. The following sections provide a comprehensive overview of its biological activity, including its metabolic pathways, therapeutic implications, and specific case studies.
- Molecular Formula : C₅H₁₄N₂O₅ (with 5 carbon atoms labeled with 13C)
- Molecular Weight : 247.19 g/mol
- CAS Number : 156968-81-9
- SMILES Notation : CC1=CN([13C@H]2[13CH2]13C@H13C@@HO2)C(=O)NC1=O
Metabolism and Biological Role
Thymidine plays a crucial role in the biosynthesis of DNA. It is phosphorylated to form deoxythymidine monophosphate (dTMP), which can further be converted into deoxythymidine triphosphate (dTTP), a building block for DNA synthesis. The enzyme thymidine kinase catalyzes this phosphorylation process, making thymidine vital for cellular proliferation and genomic stability.
Table 1: Enzymatic Pathways Involving Thymidine
Enzyme | Reaction Description |
---|---|
Thymidine Kinase | Converts thymidine to dTMP |
Thymidylate Synthase | Converts dUMP to dTMP, providing the sole de novo source |
Cytosolic Purine Nucleotidase | Converts 5-thymidylic acid to thymidine |
Cancer Research
This compound has been utilized in cancer research to trace metabolic pathways in tumor cells. For instance, studies have shown that cancer cells exhibit altered thymidine metabolism compared to normal cells. The incorporation of labeled thymidine into DNA can be measured using mass spectrometry, allowing researchers to assess the rate of DNA synthesis and cell proliferation in various cancer models.
Case Study : A study investigating the role of MTHFD2 (methylenetetrahydrofolate dehydrogenase) in acute myeloid leukemia (AML) demonstrated that inhibition of this enzyme reduced thymidine production, leading to replication stress and apoptosis in cancer cells. Supplementation with thymidine rescued the viability of these cells, indicating its critical role in maintaining DNA synthesis under stress conditions .
Metabolic Tracing
This compound is also employed in metabolic tracing studies to understand nucleotide metabolism under different physiological conditions. Research has indicated that various tumors can utilize exogenous thymidine for rapid growth, particularly under nutrient-restricted environments.
Table 2: Thymidine Utilization in Cancer Cells
Q & A
Basic Research Questions
Q. What are the established synthesis protocols for Thymidine-1',2',3',4',5'-¹³C₅, and how do isotopic purity and yield vary across methods?
- Methodological Answer : Synthesis typically involves multi-step protection/deprotection strategies. For example, thymidine derivatives are first acetylated (e.g., 3',5'-di-O-acetylation) to protect hydroxyl groups, followed by isotopic substitution via nucleophilic displacement or enzymatic methods. Purification via reversed-phase HPLC ensures >95% isotopic purity, with yields averaging 30–40% depending on reaction scale . Critical steps include nitrogen purging to prevent oxidation and rigorous solvent removal under reduced pressure to avoid side reactions .
Q. How is Thymidine-¹³C₅ quantified in biological matrices, and what validation parameters ensure assay reliability?
- Methodological Answer : Liquid chromatography-tandem mass spectrometry (LC-MS/MS) is the gold standard. Key parameters:
- Chromatography : Use a C18 column with 0.1% formic acid in water/acetonitrile gradients for baseline separation of thymidine and its analogs.
- Internal Standards : Deuterated or ¹³C₅-labeled analogs (e.g., thymidine-¹³C₅) correct for matrix effects.
- Validation : Assess linearity (1–1000 ng/mL), precision (<15% CV), and carry-over (<2% for urine, <5% for plasma). Stability tests confirm stock solutions retain >95% integrity over 2.5 months .
Q. What metabolic pathways can be traced using Thymidine-¹³C₅ in isotope labeling studies?
- Methodological Answer : Thymidine-¹³C₅ is incorporated into DNA via salvage pathways, enabling tracking of nucleotide recycling. In cancer models, it reveals metabolic flux through the pentose phosphate pathway (PPP) and Krebs cycle via ¹³C-enriched ribose and acetyl-CoA derivatives. For example, in LUAD cells, LC-MS analysis of ¹³C-labeled Sed7P and Ery4P confirms PPP activity under nutrient deprivation .
Advanced Research Questions
Q. How do researchers design isotope-tracing experiments to study thymidine salvage pathway dysregulation in mitochondrial disorders?
- Methodological Answer :
- Experimental Design : Administer Thymidine-¹³C₅ intravenously in murine models of mitochondrial neurogastrointestinal encephalomyopathy (MNGIE). Collect plasma/urine at timed intervals for LC-MS/MS analysis.
- Controls : Use wild-type mice and unlabeled thymidine controls to distinguish endogenous vs. exogenous metabolite pools.
- Data Interpretation : Quantify ¹³C enrichment in 2'-deoxyuridine (dUrd) to assess thymidine phosphorylase activity. A >20% reduction in dUrd-¹³C₅ indicates enzyme dysfunction .
Q. What analytical challenges arise from isotopic interference when using Thymidine-¹³C₅ in high-resolution metabolomics?
- Methodological Answer :
- Isotopic Crosstalk : Natural abundance ¹³C (1.1%) in unlabeled samples can mimic low-level ¹³C₅ signals. Correct using background subtraction algorithms (e.g., NoiseReduceR).
- Resolution Requirements : HRMS instruments (Orbitrap/Q-TOF) with resolving power >50,000 distinguish ¹³C₅ thymidine (m/z 243.1) from neighboring peaks.
- Validation : Spike unlabeled samples with ¹³C₅ standards to confirm specificity .
Q. How can researchers optimize reproducibility in Thymidine-¹³C₅ synthesis across laboratories?
- Methodological Answer :
- Protocol Harmonization : Standardize reaction conditions (e.g., 40°C for 36 hours in ammonia/methanol for deprotection).
- Quality Control : Batch testing via NMR (¹³C satellites at δ 60–90 ppm) and LC-MS/MS (MH⁺ ion at m/z 243.1).
- Inter-lab Calibration : Share reference materials (e.g., CIL-certified Thymidine-¹³C₅) to align purity thresholds (>98%) .
Q. What integrative approaches combine Thymidine-¹³C₅ tracing with multi-omics to study nucleotide metabolism in cancer?
- Methodological Answer :
- Workflow :
Isotope Tracing : Pulse-chase Thymidine-¹³C₅ in 3D tumor spheroids.
Transcriptomics : RNA-seq identifies upregulated salvage genes (e.g., TK1).
Metabolomics : LC-HRMS quantifies ¹³C-labeled dTTP and dUTP pools.
- Data Integration : Use pathway enrichment tools (e.g., MetaboAnalyst) to correlate thymidine uptake with PPP and TCA cycle activity. A >2-fold increase in ¹³C₅-dTTP links to chemoresistance .
Q. Safety and Best Practices
特性
IUPAC Name |
1-[(2R,4S,5R)-4-hydroxy-5-(hydroxy(113C)methyl)(2,3,4,5-13C4)oxolan-2-yl]-5-methylpyrimidine-2,4-dione | |
---|---|---|
Source | PubChem | |
URL | https://pubchem.ncbi.nlm.nih.gov | |
Description | Data deposited in or computed by PubChem | |
InChI |
InChI=1S/C10H14N2O5/c1-5-3-12(10(16)11-9(5)15)8-2-6(14)7(4-13)17-8/h3,6-8,13-14H,2,4H2,1H3,(H,11,15,16)/t6-,7+,8+/m0/s1/i2+1,4+1,6+1,7+1,8+1 | |
Source | PubChem | |
URL | https://pubchem.ncbi.nlm.nih.gov | |
Description | Data deposited in or computed by PubChem | |
InChI Key |
IQFYYKKMVGJFEH-DUGFCQTISA-N | |
Source | PubChem | |
URL | https://pubchem.ncbi.nlm.nih.gov | |
Description | Data deposited in or computed by PubChem | |
Canonical SMILES |
CC1=CN(C(=O)NC1=O)C2CC(C(O2)CO)O | |
Source | PubChem | |
URL | https://pubchem.ncbi.nlm.nih.gov | |
Description | Data deposited in or computed by PubChem | |
Isomeric SMILES |
CC1=CN(C(=O)NC1=O)[13C@H]2[13CH2][13C@@H]([13C@H](O2)[13CH2]O)O | |
Source | PubChem | |
URL | https://pubchem.ncbi.nlm.nih.gov | |
Description | Data deposited in or computed by PubChem | |
Molecular Formula |
C10H14N2O5 | |
Source | PubChem | |
URL | https://pubchem.ncbi.nlm.nih.gov | |
Description | Data deposited in or computed by PubChem | |
Molecular Weight |
247.19 g/mol | |
Source | PubChem | |
URL | https://pubchem.ncbi.nlm.nih.gov | |
Description | Data deposited in or computed by PubChem | |
試験管内研究製品の免責事項と情報
BenchChemで提示されるすべての記事および製品情報は、情報提供を目的としています。BenchChemで購入可能な製品は、生体外研究のために特別に設計されています。生体外研究は、ラテン語の "in glass" に由来し、生物体の外で行われる実験を指します。これらの製品は医薬品または薬として分類されておらず、FDAから任何の医療状態、病気、または疾患の予防、治療、または治癒のために承認されていません。これらの製品を人間または動物に体内に導入する形態は、法律により厳格に禁止されています。これらのガイドラインに従うことは、研究と実験において法的および倫理的な基準の遵守を確実にするために重要です。