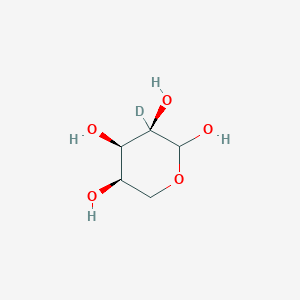
d-リボース-2-d
概要
説明
(3R,4R,5R)-3-Deuteriooxane-2,3,4,5-tetrol is a deuterated derivative of oxane, a six-membered ring compound containing oxygen. The presence of deuterium, a stable isotope of hydrogen, in the molecule makes it particularly interesting for various scientific studies, especially in the fields of chemistry and biology. This compound is often used as a model to study the effects of isotopic substitution on chemical and physical properties.
科学的研究の応用
Chemistry: (3R,4R,5R)-3-Deuteriooxane-2,3,4,5-tetrol is used as a model compound to study isotope effects in chemical reactions. It helps in understanding the kinetic isotope effect and the role of hydrogen bonding in various chemical processes.
Biology: In biological studies, this compound is used to investigate the effects of deuterium on metabolic pathways. It is also used in tracer studies to track the movement of molecules within biological systems.
Medicine: The compound is explored for its potential use in deuterium-labeled drugs. Deuterium substitution can enhance the metabolic stability of drugs, leading to longer-lasting therapeutic effects.
Industry: In the industrial sector, (3R,4R,5R)-3-Deuteriooxane-2,3,4,5-tetrol is used in the production of deuterated solvents and reagents, which are essential for various analytical techniques such as nuclear magnetic resonance (NMR) spectroscopy.
作用機序
Target of Action
D-[2-2H]ribose, also known as (3R,4R,5R)-3-Deuteriooxane-2,3,4,5-tetrol, primarily targets the enzyme Decaprenyl-phosphoryl-β-D-ribose 2’-epimerase (DprE1) which is a key enzyme implicated in the mycobacterial cell wall biosynthesis . DprE1 is involved in the synthesis of Araf molecules, which are the building blocks in the synthesis of lipoarabinomannans and arabinogalactans .
Mode of Action
It is suggested that d-[2-2h]ribose may indirectly stimulate pancreatic insulin secretion, stimulate humoral effectors causing secretion of minor, but important, amounts of insulin from tissues in the hepatic-portal pathway, and delay glucose recruitment in the liver, likely due to competition for phosphoglucomutase activity .
Biochemical Pathways
D-[2-2H]ribose is involved in various biochemical pathways. It is a component of the structure of ATP, the main energy source for cells . It is also involved in the pentose phosphate pathway (PPP), contributing to adenosine triphosphate (ATP) production and participating in nucleotide synthesis . D-[2-2H]ribose activates central carbon metabolism including glycolysis, the pentose phosphate pathway (PPP), and the tricarboxylic acid cycle (TCA cycle), increases the abundance of NADH, polarizes the electron transport chain (ETC), and elevates the proton motive force (PMF) of cells .
Pharmacokinetics
It is known that d-[2-2h]ribose can undergo phosphorylation to yield ribose-5-phosphate (r-5-p) within the cellular milieu . More research is needed to fully understand the ADME properties of D-[2-2H]ribose .
Result of Action
The molecular and cellular effects of D-[2-2H]ribose’s action are diverse. It has been shown to improve cellular processes when there is mitochondrial dysfunction . It may help recover stores of ATP in muscle cells after periods of intense exercise . Recent clinical studies have suggested a potential link between d-[2-2h]ribose metabolic disturbances and type 2 diabetes mellitus (t2dm) along with its associated complications .
Action Environment
The action, efficacy, and stability of D-[2-2H]ribose can be influenced by various environmental factors. For instance, people with diabetes who are taking medications to lower blood sugar may need to avoid supplementing with D-[2-2H]ribose, as it may lower blood sugar . More research is needed to fully understand how environmental factors influence the action of D-[2-2H]ribose.
Safety and Hazards
将来の方向性
The future directions of research into similar compounds could involve further exploration of their synthesis, characterization, and potential applications . This could include investigating their biological activity, developing new synthetic methods, and studying their physical and chemical properties in more detail.
生化学分析
Biochemical Properties
D-ribose-2-d is involved in several biochemical reactions, primarily through its role as a precursor in the synthesis of nucleotides and nucleic acids. It interacts with various enzymes, proteins, and other biomolecules. One of the key enzymes it interacts with is ribokinase, which catalyzes the phosphorylation of D-ribose-2-d to D-ribose-2-d-5-phosphate. This phosphorylated form can then enter the pentose phosphate pathway, contributing to the synthesis of nucleotides and nucleic acids .
Additionally, D-ribose-2-d interacts with enzymes involved in the non-oxidative phase of the pentose phosphate pathway, such as transketolase and transaldolase. These interactions facilitate the conversion of D-ribose-2-d-5-phosphate into other sugar phosphates, which are essential for nucleotide biosynthesis and cellular metabolism .
Cellular Effects
D-ribose-2-d influences various cellular processes, including cell signaling pathways, gene expression, and cellular metabolism. It plays a critical role in the synthesis of adenosine triphosphate (ATP), which is essential for cellular energy production . By contributing to the pentose phosphate pathway, D-ribose-2-d helps maintain the cellular redox state and supports the synthesis of nucleotides required for DNA and RNA synthesis .
Moreover, D-ribose-2-d has been shown to affect cell signaling pathways by modulating the levels of reactive oxygen species (ROS) and influencing the activity of antioxidant enzymes. This modulation can impact gene expression and cellular responses to oxidative stress .
Molecular Mechanism
At the molecular level, D-ribose-2-d exerts its effects through several mechanisms. One of the primary mechanisms is the formation of D-ribose-2-d-5-phosphate via phosphorylation by ribokinase. This phosphorylated form can then participate in the pentose phosphate pathway, contributing to the synthesis of nucleotides and nucleic acids .
D-ribose-2-d also interacts with enzymes involved in the non-oxidative phase of the pentose phosphate pathway, such as transketolase and transaldolase. These interactions facilitate the conversion of D-ribose-2-d-5-phosphate into other sugar phosphates, which are essential for nucleotide biosynthesis and cellular metabolism .
Furthermore, D-ribose-2-d can influence gene expression by modulating the levels of ROS and affecting the activity of antioxidant enzymes. This modulation can impact cellular responses to oxidative stress and contribute to the regulation of various cellular processes .
Temporal Effects in Laboratory Settings
In laboratory settings, the effects of D-ribose-2-d can vary over time. Studies have shown that D-ribose-2-d is relatively stable under physiological conditions, allowing for its sustained presence and activity in biochemical reactions . Its stability and degradation can be influenced by factors such as temperature, pH, and the presence of other reactive molecules .
Long-term studies have indicated that D-ribose-2-d can have lasting effects on cellular function, particularly in terms of energy metabolism and nucleotide synthesis . These effects are observed in both in vitro and in vivo studies, highlighting the importance of D-ribose-2-d in maintaining cellular homeostasis and supporting metabolic processes .
Dosage Effects in Animal Models
The effects of D-ribose-2-d can vary with different dosages in animal models. Studies have shown that low to moderate doses of D-ribose-2-d can enhance cellular energy production and improve metabolic function . High doses of D-ribose-2-d may lead to adverse effects, such as increased oxidative stress and disruption of cellular homeostasis .
Threshold effects have been observed, where the beneficial effects of D-ribose-2-d plateau at certain dosages, and further increases in dosage do not result in additional benefits . It is important to carefully monitor and optimize the dosage of D-ribose-2-d in animal studies to achieve the desired effects without causing toxicity .
Metabolic Pathways
D-ribose-2-d is involved in several metabolic pathways, primarily through its role in the pentose phosphate pathway. Upon phosphorylation by ribokinase, D-ribose-2-d is converted to D-ribose-2-d-5-phosphate, which can then enter the pentose phosphate pathway . This pathway is essential for the synthesis of nucleotides and nucleic acids, as well as for maintaining the cellular redox state .
D-ribose-2-d also interacts with enzymes involved in the non-oxidative phase of the pentose phosphate pathway, such as transketolase and transaldolase . These interactions facilitate the conversion of D-ribose-2-d-5-phosphate into other sugar phosphates, which are essential for nucleotide biosynthesis and cellular metabolism .
Transport and Distribution
Within cells and tissues, D-ribose-2-d is transported and distributed through various mechanisms. It can be taken up by cells via specific transporters, such as glucose transporters, which facilitate its entry into the cytoplasm . Once inside the cell, D-ribose-2-d can be phosphorylated by ribokinase and participate in metabolic pathways .
D-ribose-2-d can also interact with binding proteins that facilitate its transport and distribution within cells and tissues . These interactions can influence the localization and accumulation of D-ribose-2-d, affecting its availability for biochemical reactions and metabolic processes .
Subcellular Localization
The subcellular localization of D-ribose-2-d can impact its activity and function. Studies have shown that D-ribose-2-d is primarily localized in the cytoplasm, where it participates in metabolic pathways such as the pentose phosphate pathway . It can also be transported to other cellular compartments, such as the nucleus, where it may play a role in nucleotide synthesis and gene expression .
Targeting signals and post-translational modifications can influence the subcellular localization of D-ribose-2-d, directing it to specific compartments or organelles . These localization patterns can impact the availability and activity of D-ribose-2-d, affecting its role in cellular processes and metabolic pathways .
準備方法
Synthetic Routes and Reaction Conditions: The synthesis of (3R,4R,5R)-3-Deuteriooxane-2,3,4,5-tetrol typically involves the deuteration of oxane derivatives. One common method is the catalytic hydrogenation of oxane in the presence of deuterium gas. The reaction is usually carried out under high pressure and temperature to ensure complete deuteration.
Industrial Production Methods: In an industrial setting, the production of (3R,4R,5R)-3-Deuteriooxane-2,3,4,5-tetrol can be scaled up by using continuous flow reactors. These reactors allow for better control of reaction conditions and can handle larger volumes of reactants, making the process more efficient and cost-effective.
Types of Reactions:
Oxidation: (3R,4R,5R)-3-Deuteriooxane-2,3,4,5-tetrol can undergo oxidation reactions to form corresponding oxo derivatives. Common oxidizing agents include potassium permanganate and chromium trioxide.
Reduction: The compound can be reduced to form deuterated alcohols using reducing agents such as lithium aluminum hydride or sodium borohydride.
Substitution: Nucleophilic substitution reactions can occur at the deuterated carbon, leading to the formation of various substituted derivatives.
Common Reagents and Conditions:
Oxidation: Potassium permanganate in acidic or basic medium.
Reduction: Lithium aluminum hydride in dry ether.
Substitution: Nucleophiles such as halides or amines in the presence of a suitable catalyst.
Major Products:
Oxidation: Deuterated oxo derivatives.
Reduction: Deuterated alcohols.
Substitution: Substituted deuterated oxane derivatives.
類似化合物との比較
(3R,4R,5R)-3-Hydroxyoxane-2,3,4,5-tetrol: This compound is similar in structure but contains a hydrogen atom instead of deuterium.
(3R,4R,5R)-3-Fluorooxane-2,3,4,5-tetrol: This compound has a fluorine atom instead of deuterium, leading to different chemical properties.
(3R,4R,5R)-3-Methyloxane-2,3,4,5-tetrol: This compound contains a methyl group instead of deuterium, affecting its reactivity and stability.
Uniqueness: The uniqueness of (3R,4R,5R)-3-Deuteriooxane-2,3,4,5-tetrol lies in its isotopic substitution. The presence of deuterium provides distinct advantages in scientific research, such as enhanced stability and altered reaction kinetics. This makes it a valuable tool for studying isotope effects and developing deuterium-labeled compounds for various applications.
特性
IUPAC Name |
(3R,4R,5R)-3-deuteriooxane-2,3,4,5-tetrol | |
---|---|---|
Source | PubChem | |
URL | https://pubchem.ncbi.nlm.nih.gov | |
Description | Data deposited in or computed by PubChem | |
InChI |
InChI=1S/C5H10O5/c6-2-1-10-5(9)4(8)3(2)7/h2-9H,1H2/t2-,3-,4-,5?/m1/s1/i4D | |
Source | PubChem | |
URL | https://pubchem.ncbi.nlm.nih.gov | |
Description | Data deposited in or computed by PubChem | |
InChI Key |
SRBFZHDQGSBBOR-ZUQYETOZSA-N | |
Source | PubChem | |
URL | https://pubchem.ncbi.nlm.nih.gov | |
Description | Data deposited in or computed by PubChem | |
Canonical SMILES |
C1C(C(C(C(O1)O)O)O)O | |
Source | PubChem | |
URL | https://pubchem.ncbi.nlm.nih.gov | |
Description | Data deposited in or computed by PubChem | |
Isomeric SMILES |
[2H][C@]1([C@@H]([C@@H](COC1O)O)O)O | |
Source | PubChem | |
URL | https://pubchem.ncbi.nlm.nih.gov | |
Description | Data deposited in or computed by PubChem | |
Molecular Formula |
C5H10O5 | |
Source | PubChem | |
URL | https://pubchem.ncbi.nlm.nih.gov | |
Description | Data deposited in or computed by PubChem | |
Molecular Weight |
151.14 g/mol | |
Source | PubChem | |
URL | https://pubchem.ncbi.nlm.nih.gov | |
Description | Data deposited in or computed by PubChem | |
Q1: What is the significance of using D-[2-2H]ribose in riboflavin biosynthesis research?
A: D-[2-2H]ribose serves as a valuable isotopic tracer in studying riboflavin biosynthesis. By feeding this deuterated ribose to organisms like Ashbya gossypii, researchers can track the incorporation of deuterium into the final riboflavin molecule. This allows them to decipher the stereochemical course of the biosynthetic pathway. []
Q2: What key finding did the study using D-[2-2H]ribose reveal about riboflavin biosynthesis?
A: The study demonstrated that feeding Ashbya gossypii with D-[2-2H]ribose led to the incorporation of deuterium at the C-2' position of the ribityl side chain in the produced riboflavin. Additionally, D-[1-2H]ribose led to deuterium incorporation in the pro-R position at C-1' of the ribityl side chain. This finding effectively ruled out the Amadori rearrangement as the mechanism for converting the ribosylamino linkage to the ribitylamino linkage in riboflavin biosynthesis. Instead, the results support a mechanism involving the formation of a Schiff base followed by stereospecific reduction. []
Q3: Were there any challenges in analyzing the results from the feeding experiments with D-[2-2H]ribose?
A: Yes, interpreting the deuterium incorporation required unambiguous assignment of the 1H NMR signals for the pro-R and pro-S hydrogens at the C-1' position of both the riboflavin precursor, 6,7-dimethyl-8-ribityllumazine, and the final riboflavin molecule, along with its tetraacetate derivative. To achieve this, the researchers synthesized stereospecifically deuterated samples of these compounds as reference points for their analysis. []
Q4: Aside from riboflavin biosynthesis, are there other applications for D-[2-2H]ribose?
A: While the provided research focuses on riboflavin, deuterated sugars like D-[2-2H]ribose can be valuable tools in broader carbohydrate research. For example, they can be used to study metabolic pathways, enzyme mechanisms, and the structure and dynamics of carbohydrates in solution using techniques like NMR spectroscopy. [, ]
試験管内研究製品の免責事項と情報
BenchChemで提示されるすべての記事および製品情報は、情報提供を目的としています。BenchChemで購入可能な製品は、生体外研究のために特別に設計されています。生体外研究は、ラテン語の "in glass" に由来し、生物体の外で行われる実験を指します。これらの製品は医薬品または薬として分類されておらず、FDAから任何の医療状態、病気、または疾患の予防、治療、または治癒のために承認されていません。これらの製品を人間または動物に体内に導入する形態は、法律により厳格に禁止されています。これらのガイドラインに従うことは、研究と実験において法的および倫理的な基準の遵守を確実にするために重要です。