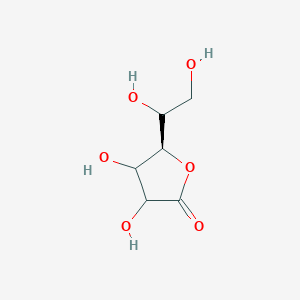
(5R)-5-(1,2-ジヒドロキシエチル)-3,4-ジヒドロキシオキソラン-2-オン
説明
“(5R)-5-(1,2-dihydroxyethyl)-3,4-dihydroxyoxolan-2-one” is a chemical compound with the molecular formula C5H8O6 . It has a molecular weight of 178.11 g/mol . The compound has a furan ring with a stereogenic center ® at the sp3 hybridized C atom .
Molecular Structure Analysis
The compound has a furan ring, which is essentially planar . The C atom bearing the dihydroxyethyl group is S. The absolute configuration is based on the precursor in the synthesis .Physical and Chemical Properties Analysis
The compound has a density of 2.0±0.1 g/cm3, a molar refractivity of 35.3±0.3 cm3, and a molar volume of 90.1±3.0 cm3 . It also has a polarizability of 14.0±0.5 10-24 cm3 and a surface tension of 140.6±3.0 dyne/cm .科学的研究の応用
触媒変換プロセス
グリーンケミストリーの分野では、この化合物はエタノールのアセトアルデヒドへの触媒的脱水素反応に役割を果たします 。このプロセスは、アセトアルデヒドの汎用的な用途と反応の高い原子経済性により重要です。エタノールを付加価値のある化学物質や燃料に変換することは、持続可能な工業プロセスへの影響を考慮して、成長している研究分野です。
有機合成
この化合物は、光誘起C-H官能基化によるキノキサリン-2(1H)-オン誘導体の合成に関与しています 。この方法は、幅広い基質範囲、高い原子経済性、穏やかな条件により注目されており、複雑な有機分子の作成のための魅力的なアプローチとなっています。
バイオテクノロジー
バイオテクノロジーの用途では、この化合物は酵素反応で使用されます。 例えば、バチルス・リケニフォルミス由来のアルカリプロテアーゼを用いた、2H-1-ベンゾピラン-2-オン誘導体の合成のための生物触媒的ドミノ反応の一部です 。これは、合成生物学における酵素の選択性と効率を高めるための化合物の有用性を示しています。
環境科学
この化合物は、植物の化学的防御機構における役割を通じて、環境科学に貢献しています。 この化合物を含むジテルペノイドの制御された水酸化は、植物の自己毒性なしに植物の防御を可能にします 。この研究は、植物がどのように化学物質を使用して、独自の成長を損なうことなく害虫から身を守るのかを理解するために不可欠です。
材料科学
材料科学では、この化合物は、SARS-CoV-2 RNA依存性RNAポリメラーゼに対する2'-置換ヌクレオチド類似体の阻害機構に関する研究の一部です 。この研究は、ウイルス複製を阻害する新しい材料やコーティングの開発に不可欠であり、公衆衛生に大きな影響を与えます。
分析化学
最後に、分析化学では、この化合物は、Mn-Fe層状複水酸化物ナノシートの触媒活性に基づく比率計測定法の開発に使用されます 。この用途は、さまざまな生物学的および化学物質を検出するためのより正確で感度の高い方法を作成するために重要です。
作用機序
将来の方向性
特性
IUPAC Name |
(5R)-5-(1,2-dihydroxyethyl)-3,4-dihydroxyoxolan-2-one | |
---|---|---|
Source | PubChem | |
URL | https://pubchem.ncbi.nlm.nih.gov | |
Description | Data deposited in or computed by PubChem | |
InChI |
InChI=1S/C6H10O6/c7-1-2(8)5-3(9)4(10)6(11)12-5/h2-5,7-10H,1H2/t2?,3?,4?,5-/m1/s1 | |
Source | PubChem | |
URL | https://pubchem.ncbi.nlm.nih.gov | |
Description | Data deposited in or computed by PubChem | |
InChI Key |
SXZYCXMUPBBULW-GHWWWWGESA-N | |
Source | PubChem | |
URL | https://pubchem.ncbi.nlm.nih.gov | |
Description | Data deposited in or computed by PubChem | |
Canonical SMILES |
C(C(C1C(C(C(=O)O1)O)O)O)O | |
Source | PubChem | |
URL | https://pubchem.ncbi.nlm.nih.gov | |
Description | Data deposited in or computed by PubChem | |
Isomeric SMILES |
C(C([C@@H]1C(C(C(=O)O1)O)O)O)O | |
Source | PubChem | |
URL | https://pubchem.ncbi.nlm.nih.gov | |
Description | Data deposited in or computed by PubChem | |
Molecular Formula |
C6H10O6 | |
Source | PubChem | |
URL | https://pubchem.ncbi.nlm.nih.gov | |
Description | Data deposited in or computed by PubChem | |
Molecular Weight |
178.14 g/mol | |
Source | PubChem | |
URL | https://pubchem.ncbi.nlm.nih.gov | |
Description | Data deposited in or computed by PubChem | |
Synthesis routes and methods
Procedure details
試験管内研究製品の免責事項と情報
BenchChemで提示されるすべての記事および製品情報は、情報提供を目的としています。BenchChemで購入可能な製品は、生体外研究のために特別に設計されています。生体外研究は、ラテン語の "in glass" に由来し、生物体の外で行われる実験を指します。これらの製品は医薬品または薬として分類されておらず、FDAから任何の医療状態、病気、または疾患の予防、治療、または治癒のために承認されていません。これらの製品を人間または動物に体内に導入する形態は、法律により厳格に禁止されています。これらのガイドラインに従うことは、研究と実験において法的および倫理的な基準の遵守を確実にするために重要です。