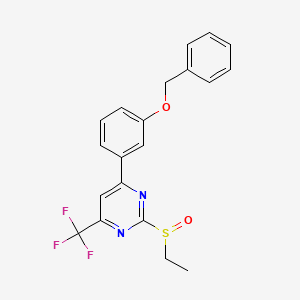
Betp
作用機序
BETPは、Gタンパク質共役受容体であるグルカゴン様ペプチド-1受容体に結合することで効果を発揮します。結合すると、this compoundは正の異種アロステリックモジュレーターとして作用し、受容体の天然のリガンドであるグルカゴン様ペプチド-1に対する反応を高めます。これにより、インスリン分泌が増加し、グルコース代謝が改善されます。 含まれる分子標的には、受容体自体と、環状アデノシン一リン酸経路などの下流のシグナル伝達経路があります .
類似の化合物との比較
This compoundは、その小分子性と経口バイオアベイラビリティのために、グルカゴン様ペプチド-1受容体アゴニストの中ではユニークです。類似の化合物には以下が含まれます。
リラグルチド: グルカゴン様ペプチド-1受容体のペプチドアゴニストで、注射によって投与されます。
セマグルチド: 注射によって投与される、もう1つのペプチドアゴニストです。
ダヌグリプロン: This compoundと類似した小分子アゴニストですが、薬物動態が異なります.
This compoundのユニークさは、経口投与が可能であることです。これにより、注射可能なペプチドアゴニストと比較して、患者のコンプライアンスが向上します .
生化学分析
Biochemical Properties
BetP transports betaine with high specificity, which under hyperosmotic conditions is accumulated in molar amounts against the concentration gradient into the cytoplasm . The transporter exploits the inward directed sodium gradient across the membrane to energize betaine transport in a stoichiometry of 2 Na+:1 betaine . It is assumed that this compound senses changes of the physical state of the membrane via its C-terminal domain .
Cellular Effects
This compound has a significant impact on cellular processes, particularly in response to osmotic stress. It senses an osmotic upshift via its osmosensory C-terminal domain and responds rapidly with a steep increase in transport rate . This rapid response helps the cell to adapt to changes in the external environment and maintain homeostasis.
Molecular Mechanism
The molecular mechanism of this compound involves a complex interplay of structural changes and interactions with other molecules. X-ray crystallography has identified four putative K+ interaction sites at the C-terminal domains of this compound . The presence of K+ results in asymmetric partial unfolding of the C-terminal domains, which is an intermediate between the downregulated state and the conformation observed in crystal structures .
Temporal Effects in Laboratory Settings
In laboratory settings, the effects of this compound can change over time. For instance, the activation of this compound is highly cooperative, with K+ binding to this compound in vivo showing a high degree of cooperativity . This suggests that the activity of this compound can be modulated by changes in the intracellular environment.
Dosage Effects in Animal Models
While specific dosage effects of this compound in animal models have not been extensively studied, it is known that the activity of this compound is regulated by the intracellular concentration of K+ . This suggests that the effects of this compound could potentially vary with different dosages of K+.
Metabolic Pathways
This compound is involved in the transport of betaine, a process that is crucial for the osmotic stress response in bacteria . This process is part of a larger metabolic pathway that helps the cell to adapt to changes in the external environment.
Transport and Distribution
This compound is a membrane transporter, and it is distributed within the cell membrane . It is thought to sense changes in the physical state of the membrane via its C-terminal domain , which may affect its distribution within the membrane.
Subcellular Localization
This compound is localized in the cell membrane, where it functions as a transporter . The C-terminal domain of this compound, which is thought to be involved in sensing osmotic stress, is attached at the membrane surface when this compound is downregulated in the absence of stress .
準備方法
合成経路と反応条件
BETPは、ピリミジンコアの形成、続いてベンジルオキシフェニル基、エチルスルフィニル基、トリフルオロメチル基の導入を含む多段階プロセスによって合成できます。合成には通常、以下が含まれます。
ピリミジンコアの形成: これは、適切な前駆体を環化を促進する条件下で反応させることで実現できます。
ベンジルオキシフェニル基の導入: このステップは、パラジウム触媒クロスカップリング反応を使用して、ベンジルオキシフェニル誘導体をピリミジンコアとカップリングすることを含みます。
エチルスルフィニル基の導入: これは、過酸化水素などの酸化剤を使用して、エチルチオ基をエチルスルフィニル基に酸化することで行うことができます。
トリフルオロメチル基の導入: このステップは、トリフルオロメチルヨージドなどの試薬を使用して、ピリミジンコアにトリフルオロメチル基を付加することを含みます.
工業生産方法
This compoundの工業生産は、同様の合成経路に従いますが、大規模生産向けに最適化されています。これには、高スループットスクリーニングと反応条件の最適化を使用して、収率と純度を最大限に引き出すことが含まれます。 プロセスには、自動合成装置と連続フロー反応器を使用し、効率とスケーラビリティを向上させることも含まれる場合があります .
化学反応の分析
反応の種類
BETPは、以下を含むさまざまな化学反応を起こします。
酸化: エチルチオ基はエチルスルフィニル基に酸化できます。
還元: エチルスルフィニル基はエチルチオ基に戻すことができます。
一般的な試薬と条件
酸化: 過酸化水素は、酸化剤として一般的に使用されます。
還元: 水素化ホウ素ナトリウムなどの還元剤を使用できます。
主要な生成物
これらの反応から形成される主要な生成物には、エチルチオ、エチルスルフィニル、および置換されたトリフルオロメチル基などの修飾された官能基を持つthis compoundの誘導体があります .
科学研究の応用
This compoundには、以下を含むいくつかの科学研究の応用があります。
化学: this compoundは、グルカゴン様ペプチド-1受容体とそのグルコース代謝における役割を研究するためのツール化合物として使用されます。
生物学: this compoundは、インスリン分泌とグルコース恒常性に関連するシグナル伝達経路を調査するために使用されます。
医学: this compoundは、2型糖尿病と肥満の治療のための潜在的な治療薬として研究されています。
科学的研究の応用
BETP has several scientific research applications, including:
Chemistry: this compound is used as a tool compound to study the glucagon-like peptide-1 receptor and its role in glucose metabolism.
Biology: this compound is used to investigate the signaling pathways involved in insulin secretion and glucose homeostasis.
Medicine: this compound is being explored as a potential therapeutic agent for the treatment of type 2 diabetes mellitus and obesity.
Industry: This compound is used in the development of new drugs targeting the glucagon-like peptide-1 receptor.
類似化合物との比較
BETP is unique among glucagon-like peptide-1 receptor agonists due to its small-molecule nature and oral bioavailability. Similar compounds include:
Liraglutide: A peptide agonist of the glucagon-like peptide-1 receptor, administered via injection.
Semaglutide: Another peptide agonist, also administered via injection.
Danuglipron: A small-molecule agonist similar to this compound, but with different pharmacokinetic properties.
This compound’s uniqueness lies in its ability to be administered orally, which improves patient compliance compared to injectable peptide agonists .
特性
IUPAC Name |
2-ethylsulfinyl-4-(3-phenylmethoxyphenyl)-6-(trifluoromethyl)pyrimidine | |
---|---|---|
Source | PubChem | |
URL | https://pubchem.ncbi.nlm.nih.gov | |
Description | Data deposited in or computed by PubChem | |
InChI |
InChI=1S/C20H17F3N2O2S/c1-2-28(26)19-24-17(12-18(25-19)20(21,22)23)15-9-6-10-16(11-15)27-13-14-7-4-3-5-8-14/h3-12H,2,13H2,1H3 | |
Source | PubChem | |
URL | https://pubchem.ncbi.nlm.nih.gov | |
Description | Data deposited in or computed by PubChem | |
InChI Key |
NTDFYGSSDDMNHI-UHFFFAOYSA-N | |
Source | PubChem | |
URL | https://pubchem.ncbi.nlm.nih.gov | |
Description | Data deposited in or computed by PubChem | |
Canonical SMILES |
CCS(=O)C1=NC(=CC(=N1)C(F)(F)F)C2=CC(=CC=C2)OCC3=CC=CC=C3 | |
Source | PubChem | |
URL | https://pubchem.ncbi.nlm.nih.gov | |
Description | Data deposited in or computed by PubChem | |
Molecular Formula |
C20H17F3N2O2S | |
Source | PubChem | |
URL | https://pubchem.ncbi.nlm.nih.gov | |
Description | Data deposited in or computed by PubChem | |
Molecular Weight |
406.4 g/mol | |
Source | PubChem | |
URL | https://pubchem.ncbi.nlm.nih.gov | |
Description | Data deposited in or computed by PubChem | |
CAS No. |
1371569-69-5 | |
Record name | 1371569-69-5 | |
Source | European Chemicals Agency (ECHA) | |
URL | https://echa.europa.eu/information-on-chemicals | |
Description | The European Chemicals Agency (ECHA) is an agency of the European Union which is the driving force among regulatory authorities in implementing the EU's groundbreaking chemicals legislation for the benefit of human health and the environment as well as for innovation and competitiveness. | |
Explanation | Use of the information, documents and data from the ECHA website is subject to the terms and conditions of this Legal Notice, and subject to other binding limitations provided for under applicable law, the information, documents and data made available on the ECHA website may be reproduced, distributed and/or used, totally or in part, for non-commercial purposes provided that ECHA is acknowledged as the source: "Source: European Chemicals Agency, http://echa.europa.eu/". Such acknowledgement must be included in each copy of the material. ECHA permits and encourages organisations and individuals to create links to the ECHA website under the following cumulative conditions: Links can only be made to webpages that provide a link to the Legal Notice page. | |
Q1: What is the primary function of BetP?
A1: this compound is a secondary active transporter that facilitates the uptake of the compatible solute glycine betaine into the cytoplasm of Corynebacterium glutamicum [, ]. This process is driven by the co-transport of sodium ions [, ].
Q2: What is the physiological role of glycine betaine accumulation in C. glutamicum?
A2: Glycine betaine acts as a compatible solute, protecting the cell from the detrimental effects of hyperosmotic stress [, ]. It helps maintain cellular turgor pressure and enzyme function under high osmolarity conditions [, , ].
Q3: How does this compound contribute to the osmotic stress response of C. glutamicum?
A3: this compound acts as both an osmosensor and an osmoregulator [, , , ]. It senses hyperosmotic conditions and responds by increasing its transport rate to rapidly accumulate glycine betaine [, ].
Q4: Does C. glutamicum rely solely on this compound for compatible solute uptake?
A4: No, C. glutamicum possesses four secondary carriers for compatible solutes: this compound, EctP, LcoP, and ProP, each with distinct substrate specificities and affinities [, ].
Q5: What is the oligomeric state of this compound?
A5: this compound functions as a homotrimer, meaning it is composed of three identical protein subunits [, , ].
Q6: Which domains of this compound are involved in sensing osmotic stress?
A6: Both the N-terminal and C-terminal cytoplasmic extensions of this compound contribute to osmosensing and regulation [, ].
Q7: What is the role of the C-terminal domain in this compound regulation?
A7: The C-terminal domain acts as a primary osmosensor, directly sensing changes in the cytoplasmic potassium ion (K+) concentration as an indicator of hyperosmotic stress [, , ].
Q8: How does the C-terminal domain transduce the osmotic signal?
A8: Upon K+ binding, the C-terminal domain undergoes conformational changes, leading to a rearrangement of the this compound trimer and activation of the transporter [, ]. This involves switching interactions between the C-terminal domain, the N-terminal domain, and the membrane lipids [, ].
Q9: What is the role of the N-terminal domain in this compound regulation?
A9: The N-terminal domain contains negatively charged clusters that interact with the positively charged C-terminal domain, modulating the activation state of this compound []. This interaction is crucial for the osmoregulatory response.
Q10: What is the significance of the five transmembrane-helix inverted-topology repeat (FIRL) fold in this compound?
A10: this compound, like other sodium-coupled transporters, adopts the FIRL fold, which is believed to be essential for the alternating access mechanism that allows for substrate transport across the membrane [].
Q11: What are the primary stimuli that activate this compound?
A11: this compound is activated by: * Increased internal K+ concentration: This is the primary signal for hyperosmotic stress response [, ].* Changes in the physical state of the membrane: The exact nature of this signal is not fully elucidated, but it is thought to involve alterations in membrane fluidity or lipid composition [, , ].
Q12: How does K+ activate this compound?
A12: K+ binds to specific sites within the C-terminal domain of this compound []. This binding is highly cooperative and triggers conformational changes that ultimately lead to transporter activation [, ].
Q13: How do changes in membrane composition affect this compound activity?
A13: The presence of negatively charged lipids, particularly phosphatidylglycerol, is crucial for this compound activity and osmoregulation [, ]. The C-terminal domain interacts with these lipids, and these interactions are modulated by K+ binding [].
Q14: Is this compound subject to regulation after initial activation?
A14: Yes, during the adaptation phase to hyperosmotic stress, this compound activity decreases despite the continued presence of a high internal K+ concentration []. This adaptation regulation is independent of K+ and might be related to changes in the hydration status of this compound or altered protein-membrane interactions [].
Q15: Does this compound exhibit any transport activity in the absence of osmotic stress?
A15: Yes, this compound displays a basal level of activity even under low osmolarity conditions. This ensures a minimal uptake of compatible solutes and allows for a rapid response upon osmotic upshift [].
Q16: What structural techniques have been employed to study this compound?
A16: Several techniques have been used to elucidate this compound structure, including:* X-ray crystallography: Provided high-resolution structures of this compound in various conformations [, ].* Cryo-electron microscopy (CryoEM): Revealed the structure of this compound in a lipid bilayer environment [].* Electron paramagnetic resonance (EPR) spectroscopy: Provided insights into the dynamics of this compound in response to different stimuli [, ].* Single-molecule FRET: Offered information about conformational changes and heterogeneity of this compound [].
Q17: What insights have structural studies provided into the mechanism of this compound activation?
A17: Structural studies, combined with biochemical and biophysical data, suggest that this compound undergoes a series of conformational changes during activation [, ]. * In the absence of K+, the C-terminal domains are located near the membrane surface, representing a downregulated state []. * Upon K+ binding, the C-terminal domains partially unfold and reorient, leading to a rearrangement of the transporter and facilitating substrate binding and transport [, ].
Q18: How does this compound compare to other osmoregulated transporters?
A18: While this compound's specific K+-dependent regulation is unique, the concept of sensing domain unfolding/refolding as a mechanism for responding to hyperosmotic stress is likely shared by other osmoregulated transporters [].
Q19: Are there any biotechnological applications of this compound or related transporters?
A19: Understanding the mechanisms of osmoregulation in bacteria like C. glutamicum has implications for optimizing their use in biotechnology [, ]. Engineering strains with enhanced osmotolerance could improve their performance in industrial processes.
試験管内研究製品の免責事項と情報
BenchChemで提示されるすべての記事および製品情報は、情報提供を目的としています。BenchChemで購入可能な製品は、生体外研究のために特別に設計されています。生体外研究は、ラテン語の "in glass" に由来し、生物体の外で行われる実験を指します。これらの製品は医薬品または薬として分類されておらず、FDAから任何の医療状態、病気、または疾患の予防、治療、または治癒のために承認されていません。これらの製品を人間または動物に体内に導入する形態は、法律により厳格に禁止されています。これらのガイドラインに従うことは、研究と実験において法的および倫理的な基準の遵守を確実にするために重要です。