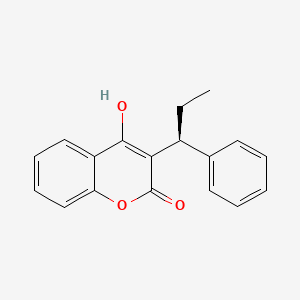
(S)-Phenprocoumon
説明
(S)-Phenprocoumon is an anticoagulant belonging to the class of coumarin derivatives. It is primarily used to prevent and treat thromboembolic disorders by inhibiting the synthesis of vitamin K-dependent clotting factors. This compound is known for its ability to reduce the risk of blood clots, making it valuable in medical settings for patients at risk of stroke, deep vein thrombosis, and pulmonary embolism.
準備方法
Synthetic Routes and Reaction Conditions: The synthesis of (S)-Phenprocoumon typically involves the condensation of 4-hydroxycoumarin with benzylideneacetone under basic conditions. The reaction proceeds through a Michael addition followed by cyclization to form the coumarin ring system. The stereochemistry of the product is controlled by using chiral catalysts or starting materials.
Industrial Production Methods: In industrial settings, the production of this compound is scaled up using similar synthetic routes but optimized for higher yields and purity. The process involves rigorous control of reaction conditions, including temperature, pH, and solvent choice, to ensure the consistent production of the desired enantiomer.
化学反応の分析
Types of Reactions: (S)-Phenprocoumon undergoes various chemical reactions, including:
Oxidation: It can be oxidized to form hydroxy derivatives.
Reduction: Reduction reactions can convert it to dihydro derivatives.
Substitution: Electrophilic substitution reactions can introduce different functional groups onto the aromatic ring.
Common Reagents and Conditions:
Oxidation: Common oxidizing agents include potassium permanganate and chromium trioxide.
Reduction: Reducing agents such as sodium borohydride or lithium aluminum hydride are used.
Substitution: Reagents like halogens, nitrating agents, and sulfonating agents are employed under controlled conditions.
Major Products:
Oxidation: Hydroxyphenprocoumon derivatives.
Reduction: Dihydro derivatives of phenprocoumon.
Substitution: Various substituted phenprocoumon derivatives depending on the reagents used.
科学的研究の応用
(S)-Phenprocoumon has a wide range of applications in scientific research:
Chemistry: It is used as a model compound to study the mechanisms of anticoagulant drugs and their interactions with other molecules.
Biology: Research on this compound helps in understanding the biological pathways involved in blood coagulation and the role of vitamin K.
Medicine: It is extensively studied for its therapeutic effects in preventing thromboembolic disorders and its pharmacokinetics and pharmacodynamics.
Industry: The compound is used in the development of new anticoagulant drugs and in quality control processes for pharmaceutical manufacturing.
作用機序
(S)-Phenprocoumon exerts its anticoagulant effects by inhibiting the enzyme vitamin K epoxide reductase. This inhibition prevents the regeneration of active vitamin K, which is essential for the synthesis of clotting factors II, VII, IX, and X. By reducing the levels of these clotting factors, this compound effectively decreases the blood’s ability to form clots. The molecular targets include the vitamin K epoxide reductase complex, and the pathways involved are the vitamin K cycle and the coagulation cascade.
類似化合物との比較
Warfarin: Another coumarin derivative with similar anticoagulant properties but different pharmacokinetics and potency.
Acenocoumarol: A coumarin anticoagulant with a shorter half-life compared to (S)-Phenprocoumon.
Dicoumarol: A naturally
生物活性
(S)-Phenprocoumon is a coumarin derivative primarily used as an oral anticoagulant. Its biological activity is closely linked to its mechanism of action, pharmacokinetics, and interactions with various substances. This article provides a comprehensive overview of the biological activity of this compound, supported by data tables, case studies, and detailed research findings.
This compound functions as an anticoagulant by inhibiting vitamin K epoxide reductase (VKOR), which is essential for the regeneration of vitamin K. This inhibition leads to a decrease in the synthesis of vitamin K-dependent clotting factors, including factors II (prothrombin), VII, IX, and X, as well as anticoagulant proteins C and S. The result is a reduced ability to form blood clots, thereby decreasing thrombogenicity.
- Key Actions:
- Inhibition of VKOR.
- Reduction in the levels of vitamin K-dependent coagulation factors.
- Decreased thrombin generation.
Pharmacokinetics
The pharmacokinetic profile of this compound is characterized by its high bioavailability and long half-life.
Parameter | Value |
---|---|
Bioavailability | ~100% |
Half-life | 5-6 days |
Protein binding | 99% |
Metabolism | Cytochrome P450 2C9 |
Clearance | Not Available |
This compound undergoes stereoselective metabolism primarily via hepatic microsomal enzymes, leading to the formation of inactive hydroxylated metabolites. The presence of polymorphisms in the CYP2C9 gene can significantly affect drug metabolism, necessitating careful monitoring and potential dosage adjustments in patients with these genetic variations .
Case-Control Study on Drug Interactions
A significant study investigated the risk of serious bleeding associated with drug interactions in patients using phenprocoumon. The study followed 246,220 patients over a total of 91,520 patient-years, identifying an incidence rate of 2.79 hospitalizations for bleeding per 100 patient-years. This highlights the importance of monitoring drug interactions in patients receiving phenprocoumon therapy .
Pharmacokinetics in Emergency Situations
A recent analysis from the RADOA registry examined pharmacokinetics in patients experiencing life-threatening bleeding or requiring urgent surgery. The study found that phenprocoumon levels had a half-life ranging from 5.27 to 5.29 days, indicating substantial variability among patients. The findings suggest that age and clinical condition significantly influence drug concentrations and outcomes .
Case Study: Influence of Liquorice Consumption
An intriguing case involved a 92-year-old female patient treated with phenprocoumon who experienced an ischemic stroke despite being within therapeutic INR ranges. Investigation revealed excessive consumption of liquorice, known to affect the pharmacokinetics of phenprocoumon by potentially reducing INR levels due to its glycyrrhizin content . This emphasizes the need for patient education regarding dietary influences on anticoagulation therapy.
Summary of Adverse Effects
The use of this compound is associated with several adverse effects:
- Bleeding complications: The most common risk associated with anticoagulants.
- Drug interactions: Significant interactions with other medications can lead to altered anticoagulation effects.
- Genetic factors: Variability in response due to genetic polymorphisms can complicate treatment.
特性
IUPAC Name |
4-hydroxy-3-[(1S)-1-phenylpropyl]chromen-2-one | |
---|---|---|
Source | PubChem | |
URL | https://pubchem.ncbi.nlm.nih.gov | |
Description | Data deposited in or computed by PubChem | |
InChI |
InChI=1S/C18H16O3/c1-2-13(12-8-4-3-5-9-12)16-17(19)14-10-6-7-11-15(14)21-18(16)20/h3-11,13,19H,2H2,1H3/t13-/m0/s1 | |
Source | PubChem | |
URL | https://pubchem.ncbi.nlm.nih.gov | |
Description | Data deposited in or computed by PubChem | |
InChI Key |
DQDAYGNAKTZFIW-ZDUSSCGKSA-N | |
Source | PubChem | |
URL | https://pubchem.ncbi.nlm.nih.gov | |
Description | Data deposited in or computed by PubChem | |
Canonical SMILES |
CCC(C1=CC=CC=C1)C2=C(C3=CC=CC=C3OC2=O)O | |
Source | PubChem | |
URL | https://pubchem.ncbi.nlm.nih.gov | |
Description | Data deposited in or computed by PubChem | |
Isomeric SMILES |
CC[C@@H](C1=CC=CC=C1)C2=C(C3=CC=CC=C3OC2=O)O | |
Source | PubChem | |
URL | https://pubchem.ncbi.nlm.nih.gov | |
Description | Data deposited in or computed by PubChem | |
Molecular Formula |
C18H16O3 | |
Source | PubChem | |
URL | https://pubchem.ncbi.nlm.nih.gov | |
Description | Data deposited in or computed by PubChem | |
DSSTOX Substance ID |
DTXSID20191141 | |
Record name | 2H-1-Benzopyran-2-one, 4-hydroxy-3-(1-phenylpropyl)-, (S)- | |
Source | EPA DSSTox | |
URL | https://comptox.epa.gov/dashboard/DTXSID20191141 | |
Description | DSSTox provides a high quality public chemistry resource for supporting improved predictive toxicology. | |
Molecular Weight |
280.3 g/mol | |
Source | PubChem | |
URL | https://pubchem.ncbi.nlm.nih.gov | |
Description | Data deposited in or computed by PubChem | |
CAS No. |
3770-63-6 | |
Record name | (-)-Phenprocoumon | |
Source | CAS Common Chemistry | |
URL | https://commonchemistry.cas.org/detail?cas_rn=3770-63-6 | |
Description | CAS Common Chemistry is an open community resource for accessing chemical information. Nearly 500,000 chemical substances from CAS REGISTRY cover areas of community interest, including common and frequently regulated chemicals, and those relevant to high school and undergraduate chemistry classes. This chemical information, curated by our expert scientists, is provided in alignment with our mission as a division of the American Chemical Society. | |
Explanation | The data from CAS Common Chemistry is provided under a CC-BY-NC 4.0 license, unless otherwise stated. | |
Record name | Phenprocoumon, (S)- | |
Source | ChemIDplus | |
URL | https://pubchem.ncbi.nlm.nih.gov/substance/?source=chemidplus&sourceid=0003770636 | |
Description | ChemIDplus is a free, web search system that provides access to the structure and nomenclature authority files used for the identification of chemical substances cited in National Library of Medicine (NLM) databases, including the TOXNET system. | |
Record name | 2H-1-Benzopyran-2-one, 4-hydroxy-3-(1-phenylpropyl)-, (S)- | |
Source | EPA DSSTox | |
URL | https://comptox.epa.gov/dashboard/DTXSID20191141 | |
Description | DSSTox provides a high quality public chemistry resource for supporting improved predictive toxicology. | |
Record name | PHENPROCOUMON, (S)- | |
Source | FDA Global Substance Registration System (GSRS) | |
URL | https://gsrs.ncats.nih.gov/ginas/app/beta/substances/3DM685514V | |
Description | The FDA Global Substance Registration System (GSRS) enables the efficient and accurate exchange of information on what substances are in regulated products. Instead of relying on names, which vary across regulatory domains, countries, and regions, the GSRS knowledge base makes it possible for substances to be defined by standardized, scientific descriptions. | |
Explanation | Unless otherwise noted, the contents of the FDA website (www.fda.gov), both text and graphics, are not copyrighted. They are in the public domain and may be republished, reprinted and otherwise used freely by anyone without the need to obtain permission from FDA. Credit to the U.S. Food and Drug Administration as the source is appreciated but not required. | |
A: (S)-Phenprocoumon inhibits the enzyme Vitamin K epoxide reductase complex subunit 1 (VKORC1) []. This enzyme is crucial for the cyclic interconversion of vitamin K, a cofactor essential for the synthesis of coagulation factors II, VII, IX, and X in the liver. By inhibiting VKORC1, this compound disrupts the production of these clotting factors, ultimately leading to an anticoagulant effect.
A: this compound is the S-enantiomer of Phenprocoumon. While the provided papers don't explicitly list the molecular formula and weight, they detail its structure as a coumarin derivative. Notably, its structure features a chiral center, leading to distinct pharmacological properties for each enantiomer [, ].
A: Research demonstrates that the (S)-enantiomer of Phenprocoumon exhibits significantly higher anticoagulant potency compared to the (R)-enantiomer [, ]. This difference in potency stems from stereoselective binding to its target, VKORC1, as well as variations in metabolism. Studies have shown that this compound is preferentially metabolized by CYP2C9, with (S)-7-hydroxylation being a major pathway [, ]. Genetic polymorphisms in CYP2C9 can significantly impact the metabolic rate of this compound, influencing its clearance and potentially affecting dosage regimens [, ].
A: Several analytical methods have been developed for the accurate quantification of this compound in biological matrices. Enantioselective liquid chromatography coupled with tandem mass spectrometry (LC-MS/MS) is a highly sensitive and specific technique used for this purpose [, ].
A: Polymorphisms in the CYP2C9 gene, particularly the 2 and 3 alleles, can significantly affect the metabolism and clearance of this compound [, , ]. Patients carrying these variant alleles may exhibit reduced metabolic capacity, potentially leading to higher drug exposure and increased bleeding risk. This highlights the importance of considering genetic factors in optimizing this compound therapy.
A: Preclinical studies utilize in vitro models such as human liver microsomes and recombinant CYP enzymes to evaluate the metabolism and drug interactions of this compound [, , ]. Animal models, such as rats, provide insights into the pharmacokinetics, pharmacodynamics, and tissue distribution of Phenprocoumon enantiomers []. Clinical trials in healthy volunteers and patients are essential for determining the drug's efficacy, safety, and optimal dosing strategies in humans [, , , ].
A: While this compound is a widely used anticoagulant, other options are available, including warfarin and acenocoumarol. These coumarin derivatives share a similar mechanism of action by inhibiting VKORC1, but they exhibit differences in potency, metabolism, and drug interaction profiles [, , , ]. The choice of anticoagulant depends on individual patient factors and clinical considerations.
試験管内研究製品の免責事項と情報
BenchChemで提示されるすべての記事および製品情報は、情報提供を目的としています。BenchChemで購入可能な製品は、生体外研究のために特別に設計されています。生体外研究は、ラテン語の "in glass" に由来し、生物体の外で行われる実験を指します。これらの製品は医薬品または薬として分類されておらず、FDAから任何の医療状態、病気、または疾患の予防、治療、または治癒のために承認されていません。これらの製品を人間または動物に体内に導入する形態は、法律により厳格に禁止されています。これらのガイドラインに従うことは、研究と実験において法的および倫理的な基準の遵守を確実にするために重要です。