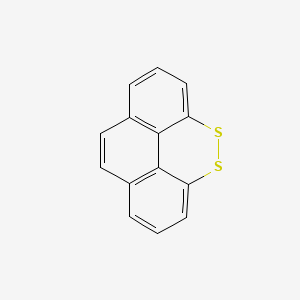
4,5-Dithiapyrene
カタログ番号 B580252
CAS番号:
129449-57-6
分子量: 240.338
InChIキー: VUTKQLWOXXWLHM-UHFFFAOYSA-N
注意: 研究専用です。人間または獣医用ではありません。
Usually In Stock
- 専門家チームからの見積もりを受け取るには、QUICK INQUIRYをクリックしてください。
- 品質商品を競争力のある価格で提供し、研究に集中できます。
説明
4,5-Dithiapyrene is a chemical compound with the formula C14H8S2 . It contains a total of 27 bonds, including 19 non-H bonds, 19 multiple bonds, 19 aromatic bonds, 4 six-membered rings, 5 ten-membered rings, and 2 twelve-membered rings .
Synthesis Analysis
The synthesis of 2,4,7,9-tetramethyl-1,6-dithiapyrene (TMDTP), a derivative of 4,5-Dithiapyrene, has been reported . The synthesis process involved five steps, and the oxidation of TMDTP was found to be facile .Molecular Structure Analysis
The molecular structure of 4,5-Dithiapyrene consists of 8 Hydrogen atoms, 14 Carbon atoms, and 2 Sulfur atoms, totaling 24 atoms . The molecule contains a total of 27 bonds, including 19 non-H bonds, 19 multiple bonds, 19 aromatic bonds, 4 six-membered rings, 5 ten-membered rings, and 2 twelve-membered rings .特性
IUPAC Name |
2,3-dithiatetracyclo[6.6.2.04,16.011,15]hexadeca-1(14),4,6,8(16),9,11(15),12-heptaene |
Source
|
---|---|---|
Source | PubChem | |
URL | https://pubchem.ncbi.nlm.nih.gov | |
Description | Data deposited in or computed by PubChem | |
InChI |
InChI=1S/C14H8S2/c1-3-9-7-8-10-4-2-6-12-14(10)13(9)11(5-1)15-16-12/h1-8H |
Source
|
Source | PubChem | |
URL | https://pubchem.ncbi.nlm.nih.gov | |
Description | Data deposited in or computed by PubChem | |
InChI Key |
VUTKQLWOXXWLHM-UHFFFAOYSA-N |
Source
|
Source | PubChem | |
URL | https://pubchem.ncbi.nlm.nih.gov | |
Description | Data deposited in or computed by PubChem | |
Canonical SMILES |
C1=CC2=C3C(=C1)SSC4=CC=CC(=C43)C=C2 |
Source
|
Source | PubChem | |
URL | https://pubchem.ncbi.nlm.nih.gov | |
Description | Data deposited in or computed by PubChem | |
Molecular Formula |
C14H8S2 |
Source
|
Source | PubChem | |
URL | https://pubchem.ncbi.nlm.nih.gov | |
Description | Data deposited in or computed by PubChem | |
Molecular Weight |
240.3 g/mol |
Source
|
Source | PubChem | |
URL | https://pubchem.ncbi.nlm.nih.gov | |
Description | Data deposited in or computed by PubChem | |
Product Name |
4,5-Dithiapyrene |
試験管内研究製品の免責事項と情報
BenchChemで提示されるすべての記事および製品情報は、情報提供を目的としています。BenchChemで購入可能な製品は、生体外研究のために特別に設計されています。生体外研究は、ラテン語の "in glass" に由来し、生物体の外で行われる実験を指します。これらの製品は医薬品または薬として分類されておらず、FDAから任何の医療状態、病気、または疾患の予防、治療、または治癒のために承認されていません。これらの製品を人間または動物に体内に導入する形態は、法律により厳格に禁止されています。これらのガイドラインに従うことは、研究と実験において法的および倫理的な基準の遵守を確実にするために重要です。
迅速なお問い合わせ
カテゴリー
最も閲覧された
4'-O-(beta-Hydroxyethyl)rutoside
13190-92-6
3-Bromo-2-methylaniline hydrochloride
16822-92-7
(+)-Norfenfluramine hydrochloride
37936-89-3
Coronene-d12
16083-32-2