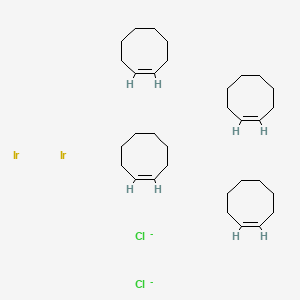
Bis(cyclooctene)iridium(I) chloride, dimer
説明
Bis(cyclooctene)iridium(I) chloride, dimer is an organoiridium compound with the formula Ir₂Cl₂(C₈H₁₄)₄. It is a yellow, air-sensitive solid that is used as a precursor to many other organoiridium compounds and catalysts . This compound is known for its role in various catalytic processes and is a valuable reagent in organometallic chemistry.
準備方法
Synthetic Routes and Reaction Conditions
Bis(cyclooctene)iridium(I) chloride, dimer can be synthesized by reacting iridium trichloride with cyclooctene in the presence of a reducing agent. The reaction typically takes place under an inert atmosphere to prevent oxidation of the iridium complex. The product is then purified by recrystallization from an appropriate solvent .
Industrial Production Methods
In industrial settings, the production of this compound involves large-scale reactions under controlled conditions. The process includes the use of high-purity starting materials and solvents to ensure the quality of the final product. The compound is often produced in batches and stored under inert gas to maintain its stability .
化学反応の分析
Types of Reactions
Bis(cyclooctene)iridium(I) chloride, dimer undergoes various types of reactions, including:
Oxidation: The compound can be oxidized to higher oxidation states of iridium.
Reduction: It can be reduced to form lower oxidation state iridium complexes.
Substitution: The cyclooctene ligands can be substituted with other ligands, such as phosphines or amines.
Common Reagents and Conditions
Oxidation: Common oxidizing agents include hydrogen peroxide and oxygen.
Reduction: Reducing agents such as sodium borohydride or lithium aluminum hydride are used.
Substitution: Ligand substitution reactions often require the presence of a coordinating solvent and may be facilitated by heating.
Major Products Formed
Oxidation: Higher oxidation state iridium complexes.
Reduction: Lower oxidation state iridium complexes.
Substitution: Various iridium complexes with different ligands.
科学的研究の応用
Bis(cyclooctene)iridium(I) chloride, dimer is used in a wide range of scientific research applications, including:
Biology: The compound is used in the study of iridium-based drugs and their interactions with biological molecules.
Medicine: Research on iridium complexes for potential therapeutic applications, including anticancer properties.
作用機序
The mechanism of action of bis(cyclooctene)iridium(I) chloride, dimer involves the coordination of the iridium center with various ligands. The iridium atom can undergo oxidative addition and reductive elimination reactions, which are key steps in many catalytic cycles. The compound’s ability to stabilize different oxidation states of iridium makes it a versatile catalyst in various chemical transformations .
類似化合物との比較
Similar Compounds
Bis(1,5-cyclooctadiene)diiridium(I) dichloride: Another iridium complex with similar catalytic properties.
Chloro(1,5-cyclooctadiene)iridium(I) dimer: A related compound used in similar catalytic applications.
Uniqueness
Bis(cyclooctene)iridium(I) chloride, dimer is unique due to its specific ligand environment, which provides distinct reactivity and stability compared to other iridium complexes. Its ability to undergo a wide range of chemical reactions and its effectiveness as a catalyst in various processes make it a valuable compound in both research and industrial applications .
生物活性
Bis(cyclooctene)iridium(I) chloride, commonly referred to as chlorobis(cyclooctene)iridium dimer, is an organoiridium compound with the molecular formula . This compound has garnered attention in various fields, particularly in catalysis and biological applications. Its unique structural properties and reactivity make it a candidate for exploring biological activities, including its potential as an anticancer agent and its interactions with biological macromolecules.
- Molecular Formula :
- Molecular Weight : 896.134 g/mol
- CAS Number : 12246-51-4
- Solubility : Soluble in organic solvents
The compound is characterized by its yellow, air-sensitive solid form and is often used as a precursor for synthesizing other iridium complexes and catalysts .
Anticancer Properties
Recent studies have indicated that bis(cyclooctene)iridium(I) chloride exhibits significant anticancer activity. For instance, research has demonstrated that iridium complexes can induce apoptosis in cancer cells through the generation of reactive oxygen species (ROS) and the activation of specific signaling pathways.
-
Mechanism of Action :
- The compound interacts with cellular components, leading to oxidative stress, which is a known pathway for inducing apoptosis in cancer cells.
- It has been shown to affect mitochondrial function and promote cell cycle arrest in cancerous cells.
-
Case Studies :
- A study published in the Journal of Organometallic Chemistry reported that iridium complexes, including bis(cyclooctene)iridium(I), displayed cytotoxic effects against various cancer cell lines, including breast and prostate cancer cells. The study noted an IC50 value indicating effective concentration levels for inducing cell death .
- Another investigation focused on the compound's ability to enhance the efficacy of established chemotherapeutics when used in combination therapies.
Interaction with Biological Macromolecules
The binding affinity of bis(cyclooctene)iridium(I) chloride to proteins and nucleic acids has been explored, revealing its potential as a bioconjugate.
-
Protein Binding Studies :
- The compound has shown a propensity to bind with serum albumin, which can influence its pharmacokinetics and distribution within biological systems.
- Binding studies using fluorescence spectroscopy indicated that the iridium complex could effectively displace other ligands from albumin, suggesting a high binding affinity .
-
Nucleic Acid Interactions :
- Research has also highlighted the ability of bis(cyclooctene)iridium(I) chloride to intercalate into DNA structures, potentially leading to mutagenic effects or serving as a delivery system for therapeutic agents.
Comparative Analysis of Biological Activities
The following table summarizes key findings from various studies regarding the biological activities of bis(cyclooctene)iridium(I) chloride compared to other iridium complexes:
Compound | Anticancer Activity | Protein Binding Affinity | DNA Interaction |
---|---|---|---|
Bis(cyclooctene)iridium(I) chloride | High (IC50 ~ µM range) | Moderate | Yes |
Iridium(III) complex | Moderate | High | Yes |
Iridium(II) complex | Low | Low | No |
特性
IUPAC Name |
cyclooctene;iridium;dichloride | |
---|---|---|
Source | PubChem | |
URL | https://pubchem.ncbi.nlm.nih.gov | |
Description | Data deposited in or computed by PubChem | |
InChI |
InChI=1S/4C8H14.2ClH.2Ir/c4*1-2-4-6-8-7-5-3-1;;;;/h4*1-2H,3-8H2;2*1H;;/p-2/b4*2-1-;;;; | |
Source | PubChem | |
URL | https://pubchem.ncbi.nlm.nih.gov | |
Description | Data deposited in or computed by PubChem | |
InChI Key |
CJJIQMGSHWWMCK-XFCUKONHSA-L | |
Source | PubChem | |
URL | https://pubchem.ncbi.nlm.nih.gov | |
Description | Data deposited in or computed by PubChem | |
Canonical SMILES |
C1CCCC=CCC1.C1CCCC=CCC1.C1CCCC=CCC1.C1CCCC=CCC1.[Cl-].[Cl-].[Ir].[Ir] | |
Source | PubChem | |
URL | https://pubchem.ncbi.nlm.nih.gov | |
Description | Data deposited in or computed by PubChem | |
Isomeric SMILES |
C1CC/C=C\CCC1.C1CC/C=C\CCC1.C1CC/C=C\CCC1.C1CC/C=C\CCC1.[Cl-].[Cl-].[Ir].[Ir] | |
Source | PubChem | |
URL | https://pubchem.ncbi.nlm.nih.gov | |
Description | Data deposited in or computed by PubChem | |
Molecular Formula |
C32H56Cl2Ir2-2 | |
Source | PubChem | |
URL | https://pubchem.ncbi.nlm.nih.gov | |
Description | Data deposited in or computed by PubChem | |
DSSTOX Substance ID |
DTXSID001045899 | |
Record name | Chlorobis(cyclooctene)iridium dimer | |
Source | EPA DSSTox | |
URL | https://comptox.epa.gov/dashboard/DTXSID001045899 | |
Description | DSSTox provides a high quality public chemistry resource for supporting improved predictive toxicology. | |
Molecular Weight |
896.1 g/mol | |
Source | PubChem | |
URL | https://pubchem.ncbi.nlm.nih.gov | |
Description | Data deposited in or computed by PubChem | |
CAS No. |
12246-51-4 | |
Record name | Chlorobis(cyclooctene)iridium dimer | |
Source | EPA DSSTox | |
URL | https://comptox.epa.gov/dashboard/DTXSID001045899 | |
Description | DSSTox provides a high quality public chemistry resource for supporting improved predictive toxicology. | |
Record name | Bis((µ-chloro)bis(cyclooctene)iridium) | |
Source | European Chemicals Agency (ECHA) | |
URL | https://echa.europa.eu/information-on-chemicals | |
Description | The European Chemicals Agency (ECHA) is an agency of the European Union which is the driving force among regulatory authorities in implementing the EU's groundbreaking chemicals legislation for the benefit of human health and the environment as well as for innovation and competitiveness. | |
Explanation | Use of the information, documents and data from the ECHA website is subject to the terms and conditions of this Legal Notice, and subject to other binding limitations provided for under applicable law, the information, documents and data made available on the ECHA website may be reproduced, distributed and/or used, totally or in part, for non-commercial purposes provided that ECHA is acknowledged as the source: "Source: European Chemicals Agency, http://echa.europa.eu/". Such acknowledgement must be included in each copy of the material. ECHA permits and encourages organisations and individuals to create links to the ECHA website under the following cumulative conditions: Links can only be made to webpages that provide a link to the Legal Notice page. | |
Q1: How does Chlorobis(cyclooctene)iridium(I) dimer interact with cerium(IV) ammonium nitrate (CAN) to facilitate the oxygen-evolution reaction (OER)? What is the proposed composition of the active catalyst?
A1: While the exact mechanism is still under investigation, the research suggests that Chlorobis(cyclooctene)iridium(I) dimer acts as a pre-catalyst in the presence of CAN. During the OER, a solid forms which exhibits catalytic activity. This solid is not simply iridium oxide, as often suggested in literature, but rather a more complex material. [] Characterization techniques revealed the presence of iridium, cerium, carbon, nitrogen, and oxygen within this catalytic material. [] Therefore, it's proposed that the active catalyst is an iridium oxide-based compound containing these elements, likely formed through the interaction of the iridium complex with CAN. [] Further research is needed to elucidate the precise structure and mechanism of this complex catalyst.
Q2: Are there any spectroscopic observations that support the formation of a catalytically active species upon mixing Chlorobis(cyclooctene)iridium(I) dimer with CAN?
A2: Yes, the research observed distinct peaks in the electronic spectra (500-750 nm) when Chlorobis(cyclooctene)iridium(I) dimer was mixed with CAN. [] Interestingly, similar peaks were observed with other iridium complexes in the presence of CAN. [] This observation led the researchers to hypothesize that a similar iridium oxide-based compound might be responsible for the OER activity across various iridium complexes when combined with CAN. []
試験管内研究製品の免責事項と情報
BenchChemで提示されるすべての記事および製品情報は、情報提供を目的としています。BenchChemで購入可能な製品は、生体外研究のために特別に設計されています。生体外研究は、ラテン語の "in glass" に由来し、生物体の外で行われる実験を指します。これらの製品は医薬品または薬として分類されておらず、FDAから任何の医療状態、病気、または疾患の予防、治療、または治癒のために承認されていません。これらの製品を人間または動物に体内に導入する形態は、法律により厳格に禁止されています。これらのガイドラインに従うことは、研究と実験において法的および倫理的な基準の遵守を確実にするために重要です。