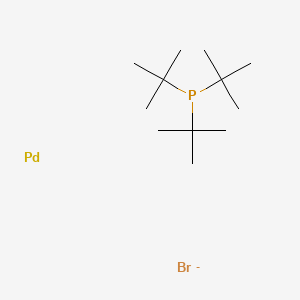
Palladium (I) tri-tert-butylphosphine bromide
説明
Palladium (I) tri-tert-butylphosphine bromide:
作用機序
Target of Action
Palladium (I) tri-tert-butylphosphine bromide primarily targets aryl chloride and sterically hindered or electron-rich aryl/vinyl bromides and iodides . These are the key components in various coupling reactions, including Suzuki coupling, Negishi coupling, and Buchwald-Hartwig amination reactions .
Mode of Action
The compound contains bulky, electron-rich tertiary phosphine ligands . In a palladium-catalyzed cross-coupling reaction, these ligands promote the oxidative addition as they can stabilize higher oxidation states . Reductive elimination is also facilitated because of the bulky ligands . This makes the compound especially active in difficult aminations .
Biochemical Pathways
The compound is involved in various biochemical pathways, including the Suzuki, Negishi, and Buchwald-Hartwig amination reactions . It is also used in Stille reactions of aryl halides . These reactions are crucial for the formation of carbon-carbon, carbon-nitrogen, and nitrogen-sulfur bonds .
Pharmacokinetics
It is known that the compound is sensitive to air and must be manipulated in a glove box or under inert gas . It is also known to be soluble in benzene and toluene, but insoluble in alcohols and water .
Result of Action
The compound’s action results in the formation of new bonds in the target molecules. For example, it can catalyze the formation of carbon-sulfur bonds . It can also catalyze the diastereoselective arylation of 4-substituted cyclohexyl esters .
Action Environment
The action of this compound is influenced by environmental factors. For instance, it must be stored under inert gas and protected from moisture . It is also sensitive to the presence of air . These factors can influence the compound’s action, efficacy, and stability.
生化学分析
Biochemical Properties
Palladium (I) tri-tert-butylphosphine bromide is known to be a catalyst for various organic reactions . It can activate aryl chloride and sterically hindered or electron-rich aryl/vinyl bromides and iodides . It is especially active in difficult aminations . The compound interacts with these biomolecules, facilitating the formation of carbon-carbon, carbon-nitrogen, and carbon-sulfur bonds .
Molecular Mechanism
This compound acts as a catalyst in biochemical reactions, facilitating the formation of bonds between biomolecules . It can activate aryl chloride and sterically hindered or electron-rich aryl/vinyl bromides and iodides . This suggests that it may exert its effects at the molecular level through binding interactions with these biomolecules.
Temporal Effects in Laboratory Settings
It is known to be air-sensitive and should be stored in an inert atmosphere, under -20°C .
準備方法
Synthetic Routes and Reaction Conditions: Palladium (I) tri-tert-butylphosphine bromide is typically synthesized by reacting tri-tert-butylphosphine with palladium chloride. The reaction involves the following steps:
- Dissolving tri-tert-butylphosphine in an organic solvent.
- Adding palladium chloride to the solution.
- Allowing the reaction to proceed under controlled conditions to form the desired product[1][1].
化学反応の分析
Types of Reactions: Palladium (I) tri-tert-butylphosphine bromide undergoes several types of reactions, including:
Oxidation: It can be oxidized to higher oxidation states of palladium.
Reduction: It can be reduced to lower oxidation states or elemental palladium.
Substitution: It participates in substitution reactions where ligands are exchanged.
Common Reagents and Conditions:
Oxidation: Common oxidizing agents include oxygen and peroxides.
Reduction: Reducing agents such as hydrogen gas or hydrides are used.
Substitution: Various ligands, including phosphines and halides, can be used under controlled conditions.
Major Products:
Oxidation: Higher oxidation state palladium compounds.
Reduction: Lower oxidation state palladium compounds or elemental palladium.
Substitution: New palladium complexes with different ligands.
科学的研究の応用
Chemistry: Palladium (I) tri-tert-butylphosphine bromide is widely used as a catalyst in organic synthesis. It facilitates various coupling reactions, including Suzuki coupling, Negishi coupling, and Buchwald-Hartwig amination. These reactions are essential for forming carbon-carbon and carbon-nitrogen bonds .
Biology and Medicine: In biological research, this compound is used to study palladium’s interactions with biomolecules.
Industry: Industrially, it is used in the production of fine chemicals and pharmaceuticals. Its catalytic properties make it valuable for large-scale synthesis of complex organic molecules .
類似化合物との比較
- Palladium (II) acetate
- Palladium (II) chloride
- Palladium (0) tetrakis(triphenylphosphine)
Comparison: Palladium (I) tri-tert-butylphosphine bromide is unique due to its specific ligand environment, which provides distinct catalytic properties. Compared to palladium (II) acetate and palladium (II) chloride, it offers better solubility in organic solvents and higher stability under reaction conditions. Palladium (0) tetrakis(triphenylphosphine) is another widely used catalyst, but it differs in its oxidation state and ligand structure .
特性
IUPAC Name |
palladium;tritert-butylphosphane;bromide | |
---|---|---|
Source | PubChem | |
URL | https://pubchem.ncbi.nlm.nih.gov | |
Description | Data deposited in or computed by PubChem | |
InChI |
InChI=1S/C12H27P.BrH.Pd/c1-10(2,3)13(11(4,5)6)12(7,8)9;;/h1-9H3;1H;/p-1 | |
Source | PubChem | |
URL | https://pubchem.ncbi.nlm.nih.gov | |
Description | Data deposited in or computed by PubChem | |
InChI Key |
WIADNXKBAGKNOT-UHFFFAOYSA-M | |
Source | PubChem | |
URL | https://pubchem.ncbi.nlm.nih.gov | |
Description | Data deposited in or computed by PubChem | |
Canonical SMILES |
CC(C)(C)P(C(C)(C)C)C(C)(C)C.[Br-].[Pd] | |
Source | PubChem | |
URL | https://pubchem.ncbi.nlm.nih.gov | |
Description | Data deposited in or computed by PubChem | |
Molecular Formula |
C12H27BrPPd- | |
Source | PubChem | |
URL | https://pubchem.ncbi.nlm.nih.gov | |
Description | Data deposited in or computed by PubChem | |
DSSTOX Substance ID |
DTXSID20747905 | |
Record name | palladium;tritert-butylphosphane;bromide | |
Source | EPA DSSTox | |
URL | https://comptox.epa.gov/dashboard/DTXSID20747905 | |
Description | DSSTox provides a high quality public chemistry resource for supporting improved predictive toxicology. | |
Molecular Weight |
388.64 g/mol | |
Source | PubChem | |
URL | https://pubchem.ncbi.nlm.nih.gov | |
Description | Data deposited in or computed by PubChem | |
CAS No. |
185812-86-6 | |
Record name | palladium;tritert-butylphosphane;bromide | |
Source | EPA DSSTox | |
URL | https://comptox.epa.gov/dashboard/DTXSID20747905 | |
Description | DSSTox provides a high quality public chemistry resource for supporting improved predictive toxicology. | |
Record name | Bromo(tri-tert-butylphosphine)palladium(I) dimer | |
Source | European Chemicals Agency (ECHA) | |
URL | https://echa.europa.eu/information-on-chemicals | |
Description | The European Chemicals Agency (ECHA) is an agency of the European Union which is the driving force among regulatory authorities in implementing the EU's groundbreaking chemicals legislation for the benefit of human health and the environment as well as for innovation and competitiveness. | |
Explanation | Use of the information, documents and data from the ECHA website is subject to the terms and conditions of this Legal Notice, and subject to other binding limitations provided for under applicable law, the information, documents and data made available on the ECHA website may be reproduced, distributed and/or used, totally or in part, for non-commercial purposes provided that ECHA is acknowledged as the source: "Source: European Chemicals Agency, http://echa.europa.eu/". Such acknowledgement must be included in each copy of the material. ECHA permits and encourages organisations and individuals to create links to the ECHA website under the following cumulative conditions: Links can only be made to webpages that provide a link to the Legal Notice page. | |
Q1: What makes Palladium(I) tri-tert-butylphosphine bromide a suitable catalyst for aminating hindered anilines?
A1: Traditional palladium catalysts often struggle with sterically hindered substrates like N-alkyl-substituted anilines, leading to low reaction yields. Palladium(I) tri-tert-butylphosphine bromide, denoted as [Pd(μ-Br)(t-Bu3P)]2, exhibits excellent catalytic activity even with these challenging substrates. [] The bulky tri-tert-butylphosphine ligands likely play a crucial role in facilitating the oxidative addition of aryl bromides to the palladium center, even when bulky anilines are involved.
Q2: What are the advantages of using [Pd(μ-Br)(t-Bu3P)]2 compared to other Palladium catalysts for this reaction?
A2: [Pd(μ-Br)(t-Bu3P)]2 offers several advantages over other palladium catalysts for the amination of aryl bromides with hindered anilines. Firstly, it is commercially available and easy to handle. [] Secondly, it demonstrates high catalytic activity, allowing for efficient coupling even with challenging substrates. This translates to potentially milder reaction conditions and higher yields compared to other catalytic systems.
試験管内研究製品の免責事項と情報
BenchChemで提示されるすべての記事および製品情報は、情報提供を目的としています。BenchChemで購入可能な製品は、生体外研究のために特別に設計されています。生体外研究は、ラテン語の "in glass" に由来し、生物体の外で行われる実験を指します。これらの製品は医薬品または薬として分類されておらず、FDAから任何の医療状態、病気、または疾患の予防、治療、または治癒のために承認されていません。これらの製品を人間または動物に体内に導入する形態は、法律により厳格に禁止されています。これらのガイドラインに従うことは、研究と実験において法的および倫理的な基準の遵守を確実にするために重要です。