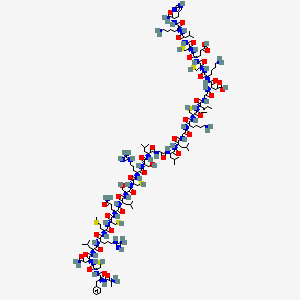
Leiurotoxin I
- 専門家チームからの見積もりを受け取るには、QUICK INQUIRYをクリックしてください。
- 品質商品を競争力のある価格で提供し、研究に集中できます。
説明
準備方法
Synthetic Routes and Reaction Conditions: Leiurotoxin I can be synthesized chemically through solid-phase peptide synthesis. This method involves the sequential addition of amino acids to a growing peptide chain anchored to a solid resin . The synthesis is typically carried out under controlled conditions to ensure the correct folding and formation of disulfide bonds, which are crucial for the biological activity of the peptide .
Industrial Production Methods: While industrial-scale production of this compound is not common, the peptide can be produced in large quantities using recombinant DNA technology. This involves inserting the gene encoding this compound into a suitable expression system, such as Escherichia coli, and inducing the production of the peptide .
化学反応の分析
Types of Reactions: Leiurotoxin I primarily undergoes oxidation and reduction reactions, particularly involving its disulfide bonds. These reactions are essential for the proper folding and stability of the peptide .
Common Reagents and Conditions:
Oxidation: Hydrogen peroxide or iodine can be used to oxidize the thiol groups of cysteine residues to form disulfide bonds.
Reduction: Dithiothreitol or β-mercaptoethanol can reduce disulfide bonds to free thiol groups.
Major Products: The major products of these reactions are the oxidized and reduced forms of this compound, which differ in their biological activity and stability .
科学的研究の応用
Leiurotoxin I has a wide range of applications in scientific research:
Neuropharmacology: It is used to study the function of small-conductance calcium-activated potassium channels in neurons.
Muscle Physiology: this compound helps in understanding the role of these channels in muscle contraction and relaxation.
Drug Development: The peptide serves as a lead compound for developing new drugs targeting potassium channels.
Toxin Research: It is used to explore the structure-function relationships of scorpion toxins and their interactions with ion channels.
作用機序
Leiurotoxin I is similar to other scorpion toxins such as apamin and charybdotoxin, which also target potassium channels . this compound is unique in its specificity for small-conductance calcium-activated potassium channels, whereas apamin targets a different subset of these channels, and charybdotoxin affects large-conductance channels . This specificity makes this compound a valuable tool for studying the distinct physiological roles of these channels .
類似化合物との比較
- Apamin
- Charybdotoxin
- Iberiotoxin
特性
IUPAC Name |
(4S)-4-[[(2R)-2-[[(2S)-6-amino-2-[[(2S)-2-[[2-[[(2S,3S)-2-[[(2R)-2-[[(2S)-6-amino-2-[[2-[[(2S)-2-[[(2S)-2-[[2-[[(2S)-2-[[(2S)-2-[[(2S)-2-[[(2R)-2-[[(2S)-2-[[(2S)-2-[[(2S)-5-amino-2-[[(2R)-2-[[(2S)-2-[[(2S)-2-[[(2S)-2-[[(2S)-4-amino-2-[[(2R)-2-[[(2S)-2-[[(2S)-2-aminopropanoyl]amino]-3-phenylpropanoyl]amino]-3-sulfanylpropanoyl]amino]-4-oxobutanoyl]amino]-4-methylpentanoyl]amino]-5-carbamimidamidopentanoyl]amino]-4-methylsulfanylbutanoyl]amino]-3-sulfanylpropanoyl]amino]-5-oxopentanoyl]amino]-4-methylpentanoyl]amino]-3-hydroxypropanoyl]amino]-3-sulfanylpropanoyl]amino]-5-carbamimidamidopentanoyl]amino]-3-hydroxypropanoyl]amino]-4-methylpentanoyl]amino]acetyl]amino]-4-methylpentanoyl]amino]-4-methylpentanoyl]amino]acetyl]amino]hexanoyl]amino]-3-sulfanylpropanoyl]amino]-3-methylpentanoyl]amino]acetyl]amino]-3-carboxypropanoyl]amino]hexanoyl]amino]-3-sulfanylpropanoyl]amino]-5-[[(2R)-1-[[(2S)-1-[[(2S)-6-amino-1-[[(2S)-1-amino-3-(1H-imidazol-4-yl)-1-oxopropan-2-yl]amino]-1-oxohexan-2-yl]amino]-3-methyl-1-oxobutan-2-yl]amino]-1-oxo-3-sulfanylpropan-2-yl]amino]-5-oxopentanoic acid |
Source
|
---|---|---|
Source | PubChem | |
URL | https://pubchem.ncbi.nlm.nih.gov | |
Description | Data deposited in or computed by PubChem | |
InChI |
InChI=1S/C142H243N45O39S7/c1-17-75(14)112(139(225)159-59-108(194)163-95(55-110(197)198)130(216)164-80(32-22-25-42-144)121(207)180-98(62-227)135(221)168-85(37-39-109(195)196)123(209)183-102(66-231)137(223)186-111(74(12)13)140(226)170-81(33-23-26-43-145)119(205)171-87(113(149)199)53-78-56-154-68-160-78)187-138(224)103(67-232)182-117(203)79(31-21-24-41-143)161-106(192)57-157-115(201)88(47-69(2)3)173-125(211)90(49-71(6)7)162-107(193)58-158-116(202)89(48-70(4)5)174-131(217)96(60-188)178-120(206)83(35-28-45-156-142(152)153)166-133(219)100(64-229)185-132(218)97(61-189)179-127(213)92(51-73(10)11)175-122(208)84(36-38-104(147)190)167-134(220)99(63-228)181-124(210)86(40-46-233-16)169-118(204)82(34-27-44-155-141(150)151)165-126(212)91(50-72(8)9)176-129(215)94(54-105(148)191)177-136(222)101(65-230)184-128(214)93(172-114(200)76(15)146)52-77-29-19-18-20-30-77/h18-20,29-30,56,68-76,79-103,111-112,188-189,227-232H,17,21-28,31-55,57-67,143-146H2,1-16H3,(H2,147,190)(H2,148,191)(H2,149,199)(H,154,160)(H,157,201)(H,158,202)(H,159,225)(H,161,192)(H,162,193)(H,163,194)(H,164,216)(H,165,212)(H,166,219)(H,167,220)(H,168,221)(H,169,204)(H,170,226)(H,171,205)(H,172,200)(H,173,211)(H,174,217)(H,175,208)(H,176,215)(H,177,222)(H,178,206)(H,179,213)(H,180,207)(H,181,210)(H,182,203)(H,183,209)(H,184,214)(H,185,218)(H,186,223)(H,187,224)(H,195,196)(H,197,198)(H4,150,151,155)(H4,152,153,156)/t75-,76-,79-,80-,81-,82-,83-,84-,85-,86-,87-,88-,89-,90-,91-,92-,93-,94-,95-,96-,97-,98-,99-,100-,101-,102-,103-,111-,112-/m0/s1 |
Source
|
Source | PubChem | |
URL | https://pubchem.ncbi.nlm.nih.gov | |
Description | Data deposited in or computed by PubChem | |
InChI Key |
MXWDLLUGULWYIQ-BFRWRHKQSA-N |
Source
|
Source | PubChem | |
URL | https://pubchem.ncbi.nlm.nih.gov | |
Description | Data deposited in or computed by PubChem | |
Canonical SMILES |
CCC(C)C(C(=O)NCC(=O)NC(CC(=O)O)C(=O)NC(CCCCN)C(=O)NC(CS)C(=O)NC(CCC(=O)O)C(=O)NC(CS)C(=O)NC(C(C)C)C(=O)NC(CCCCN)C(=O)NC(CC1=CNC=N1)C(=O)N)NC(=O)C(CS)NC(=O)C(CCCCN)NC(=O)CNC(=O)C(CC(C)C)NC(=O)C(CC(C)C)NC(=O)CNC(=O)C(CC(C)C)NC(=O)C(CO)NC(=O)C(CCCNC(=N)N)NC(=O)C(CS)NC(=O)C(CO)NC(=O)C(CC(C)C)NC(=O)C(CCC(=O)N)NC(=O)C(CS)NC(=O)C(CCSC)NC(=O)C(CCCNC(=N)N)NC(=O)C(CC(C)C)NC(=O)C(CC(=O)N)NC(=O)C(CS)NC(=O)C(CC2=CC=CC=C2)NC(=O)C(C)N |
Source
|
Source | PubChem | |
URL | https://pubchem.ncbi.nlm.nih.gov | |
Description | Data deposited in or computed by PubChem | |
Isomeric SMILES |
CC[C@H](C)[C@@H](C(=O)NCC(=O)N[C@@H](CC(=O)O)C(=O)N[C@@H](CCCCN)C(=O)N[C@@H](CS)C(=O)N[C@@H](CCC(=O)O)C(=O)N[C@@H](CS)C(=O)N[C@@H](C(C)C)C(=O)N[C@@H](CCCCN)C(=O)N[C@@H](CC1=CNC=N1)C(=O)N)NC(=O)[C@H](CS)NC(=O)[C@H](CCCCN)NC(=O)CNC(=O)[C@H](CC(C)C)NC(=O)[C@H](CC(C)C)NC(=O)CNC(=O)[C@H](CC(C)C)NC(=O)[C@H](CO)NC(=O)[C@H](CCCNC(=N)N)NC(=O)[C@H](CS)NC(=O)[C@H](CO)NC(=O)[C@H](CC(C)C)NC(=O)[C@H](CCC(=O)N)NC(=O)[C@H](CS)NC(=O)[C@H](CCSC)NC(=O)[C@H](CCCNC(=N)N)NC(=O)[C@H](CC(C)C)NC(=O)[C@H](CC(=O)N)NC(=O)[C@H](CS)NC(=O)[C@H](CC2=CC=CC=C2)NC(=O)[C@H](C)N |
Source
|
Source | PubChem | |
URL | https://pubchem.ncbi.nlm.nih.gov | |
Description | Data deposited in or computed by PubChem | |
Molecular Formula |
C142H243N45O39S7 |
Source
|
Source | PubChem | |
URL | https://pubchem.ncbi.nlm.nih.gov | |
Description | Data deposited in or computed by PubChem | |
Molecular Weight |
3429.2 g/mol |
Source
|
Source | PubChem | |
URL | https://pubchem.ncbi.nlm.nih.gov | |
Description | Data deposited in or computed by PubChem | |
CAS No. |
142948-19-4 |
Source
|
Record name | 142948-19-4 | |
Source | European Chemicals Agency (ECHA) | |
URL | https://echa.europa.eu/information-on-chemicals | |
Description | The European Chemicals Agency (ECHA) is an agency of the European Union which is the driving force among regulatory authorities in implementing the EU's groundbreaking chemicals legislation for the benefit of human health and the environment as well as for innovation and competitiveness. | |
Explanation | Use of the information, documents and data from the ECHA website is subject to the terms and conditions of this Legal Notice, and subject to other binding limitations provided for under applicable law, the information, documents and data made available on the ECHA website may be reproduced, distributed and/or used, totally or in part, for non-commercial purposes provided that ECHA is acknowledged as the source: "Source: European Chemicals Agency, http://echa.europa.eu/". Such acknowledgement must be included in each copy of the material. ECHA permits and encourages organisations and individuals to create links to the ECHA website under the following cumulative conditions: Links can only be made to webpages that provide a link to the Legal Notice page. | |
試験管内研究製品の免責事項と情報
BenchChemで提示されるすべての記事および製品情報は、情報提供を目的としています。BenchChemで購入可能な製品は、生体外研究のために特別に設計されています。生体外研究は、ラテン語の "in glass" に由来し、生物体の外で行われる実験を指します。これらの製品は医薬品または薬として分類されておらず、FDAから任何の医療状態、病気、または疾患の予防、治療、または治癒のために承認されていません。これらの製品を人間または動物に体内に導入する形態は、法律により厳格に禁止されています。これらのガイドラインに従うことは、研究と実験において法的および倫理的な基準の遵守を確実にするために重要です。