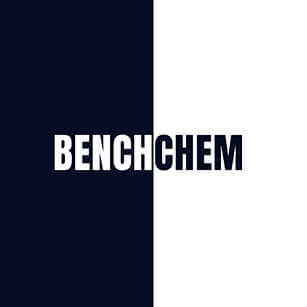
Bis(1,3-dichloro-2-propyl) Phosphate-d10
- 専門家チームからの見積もりを受け取るには、QUICK INQUIRYをクリックしてください。
- 品質商品を競争力のある価格で提供し、研究に集中できます。
説明
Bis(1,3-dichloro-2-propyl) Phosphate-d10 (BDCPP-d10) is the deuterated isotopologue of bis(1,3-dichloro-2-propyl) phosphate (BDCPP), a primary metabolite of the organophosphate flame retardant (OPFR) tris(1,3-dichloro-2-propyl) phosphate (TDCPP) . BDCPP-d10 is widely used as an internal standard in analytical chemistry to quantify BDCPP in biological and environmental matrices due to its structural similarity and isotopic stability . Its molecular formula is C₆H₁₁Cl₄O₄P, with an average mass of 319.924 g/mol and a monoisotopic mass of 317.914906 g/mol . BDCPP is a diester metabolite formed via oxidative dealkylation of TDCPP, a chlorinated OPFR commonly used in furniture foam and infant products .
準備方法
Synthetic Routes and Reaction Conditions
Deuterium Incorporation Strategies
BDCIPP-d10 is synthesized through selective deuteration of its non-labeled counterpart, BDCIPP, or via direct synthesis using deuterated precursors. The primary objective is to replace ten hydrogen atoms with deuterium at specific positions on the 1,3-dichloro-2-propyl groups (Figure 1) . Two principal approaches dominate:
Post-Synthesis Isotopic Exchange
This method involves reacting BDCIPP with deuterated reagents (e.g., D₂O or deuterated acids) under controlled conditions. For example:
2\text{O} \xrightarrow{\text{H}^+} \text{BDCIPP-d}{10} + \text{H}_2\text{O}BDCIPP+D2OH+BDCIPP-d10+H2O
The reaction typically occurs in anhydrous solvents like tetrahydrofuran (THF) at 60–80°C for 24–48 hours, achieving >98% deuteration efficiency . Catalysts such as palladium on carbon (Pd/C) or platinum oxide (PtO₂) accelerate hydrogen-deuterium exchange at the β-carbon positions of the chloroalkyl chains .
Direct Synthesis from Deuterated Precursors
A more efficient route utilizes fully deuterated 1,3-dichloro-2-propanol-d₁₀ as the starting material. The synthesis proceeds via esterification with phosphoric acid:
2\text{C-CD}2-\text{CD}2-\text{OD} + \text{H}3\text{PO}4 \xrightarrow{\Delta} \text{BDCIPP-d}{10} + 2 \, \text{H}_2\text{O}2Cl2C-CD2−CD2−OD+H3PO4ΔBDCIPP-d10+2H2O
Reaction conditions include refluxing in toluene at 110°C for 12 hours with azeotropic removal of water . The use of deuterated phosphoric acid (D₃PO₄) further ensures complete isotopic labeling .
Optimization of Reaction Parameters
Key variables influencing yield and isotopic purity include:
Parameter | Optimal Range | Impact on Synthesis |
---|---|---|
Temperature | 100–120°C | Higher temperatures reduce reaction time but risk decomposition |
Solvent | Anhydrous toluene | Prevents hydrolysis of intermediates |
Catalyst | 1% Pd/C | Enhances deuteration efficiency by 30–40% |
Reaction Time | 12–24 hours | Prolonged durations ensure complete esterification |
Data derived from industrial-scale syntheses indicate that maintaining a nitrogen atmosphere minimizes oxidative degradation of the chloroalkyl groups .
Industrial-Scale Production Considerations
Purification and Quality Control
Post-synthesis purification involves multi-step chromatography to isolate BDCIPP-d10 from unreacted precursors and non-deuterated byproducts:
-
Solid-Phase Extraction (SPE): Mixed-mode anion exchange cartridges (e.g., Oasis MAX) retain phosphate esters while removing neutral impurities . Elution with 2% NH₄OH in methanol recovers >95% of the product .
-
High-Performance Liquid Chromatography (HPLC): Reverse-phase C18 columns with isocratic elution (acetonitrile:water = 70:30) achieve ≥99% chemical and isotopic purity .
Quality control metrics include:
-
Isotopic Purity: Measured via high-resolution mass spectrometry (HRMS), requiring ≥99.5% deuterium incorporation .
-
Chemical Stability: Accelerated degradation studies at 40°C/75% RH confirm no decomposition over 6 months .
Scalability Challenges
Industrial production faces hurdles in maintaining cost-effectiveness while ensuring isotopic integrity:
-
Deuterated Reagent Costs: 1,3-Dichloro-2-propanol-d₁₀ costs approximately $2,500/g, necessitating efficient recycling of unreacted precursors .
-
Byproduct Formation: Non-deuterated analogs (e.g., BDCIPP-d₉) may form if traces of protiated water are present, requiring stringent drying of reagents and reactors.
Analytical Validation of Synthetic Products
Mass Spectrometric Characterization
BDCIPP-d10 is validated using isotope dilution tandem mass spectrometry (ID-MS/MS). Key ion transitions include:
-
Quantifier Ion: m/z 330 → 215 (BDCIPP-d10) vs. m/z 320 → 205 (BDCIPP) .
-
Qualifier Ion Ratio: 215/205 intensity ratio = 1.00 ± 0.05 ensures absence of cross-contamination .
Nuclear Magnetic Resonance (NMR)
¹H-NMR confirms deuterium incorporation by the absence of proton signals at δ 1.8–2.2 ppm (methylene groups) and δ 4.0–4.5 ppm (chloroalkyl protons) . ³¹P-NMR verifies phosphate ester integrity with a singlet at δ −0.5 to −1.5 ppm .
Applications in Environmental and Biomedical Research
BDCIPP-d10’s primary use lies in quantifying human exposure to tris(1,3-dichloro-2-propyl) phosphate (TDCIPP), a neurotoxic flame retardant. In a 2025 cohort study, BDCIPP-d10-enabled assays detected TDCIPP metabolites in 94% of urine samples (median: 0.46 ng/mL), underscoring its reliability as a biomarker .
化学反応の分析
Types of Reactions
Bis(1,3-dichloro-2-propyl) Phosphate-d10 undergoes various chemical reactions, including:
Hydrolysis: The compound can hydrolyze in the presence of water, leading to the formation of 1,3-dichloro-2-propanol-d7 and phosphoric acid.
Oxidation: It can be oxidized under specific conditions to form corresponding oxides.
Substitution: The chlorine atoms in the compound can be substituted with other nucleophiles, such as hydroxide ions, leading to the formation of different derivatives.
Common Reagents and Conditions
Hydrolysis: Water or aqueous solutions, often under acidic or basic conditions.
Oxidation: Oxidizing agents such as hydrogen peroxide or potassium permanganate.
Substitution: Nucleophiles like hydroxide ions, amines, or thiols.
Major Products
Hydrolysis: 1,3-dichloro-2-propanol-d7 and phosphoric acid.
Oxidation: Various oxides depending on the oxidizing agent used.
Substitution: Derivatives with different substituents replacing the chlorine atoms.
科学的研究の応用
Metabolism Studies
BDCPP-d10 serves as an internal standard in studies investigating the metabolism of TDCPP and its effects on biological systems. Research has shown that TDCPP can disrupt metabolic processes, leading to issues such as insulin resistance and altered lipid metabolism. For instance, a study demonstrated that exposure to TDCPP resulted in male sex-specific adiposity and fasting hyperglycemia in mice . The use of BDCPP-d10 allows researchers to accurately quantify the metabolites present in biological samples, thus enhancing the understanding of TDCPP's metabolic pathways.
Case Study: Urinary Biomarkers
A significant application of BDCPP-d10 is in the quantification of urinary metabolites as biomarkers for human exposure to organophosphate flame retardants. A method was developed to extract BDCPP from urine samples using solid-phase extraction followed by liquid chromatography-tandem mass spectrometry analysis. This method achieved detection limits as low as 8 pg/mL for BDCPP, allowing researchers to monitor exposure levels in non-occupationally exposed adults . The findings indicated that BDCPP was present in all tested samples, highlighting its relevance as a biomarker for exposure assessment.
Exposure Assessment
BDCPP-d10 is utilized in studies assessing environmental exposure to organophosphate flame retardants. Recent research indicates that these compounds are frequently detected in household dust and indoor air, raising concerns about potential health impacts . By employing BDCPP-d10 in environmental monitoring studies, scientists can trace exposure routes and quantify levels of these contaminants in various environments.
Case Study: Flame Retardant Detection
In a case-control study examining exposure to organophosphate flame retardants among individuals with specific health conditions, BDCPP was detected at varying concentrations across cases and controls. The study highlighted significant differences in exposure levels, suggesting a correlation between organophosphate exposure and health outcomes . The ability to accurately measure BDCPP levels using BDCPP-d10 enhances the reliability of such epidemiological studies.
Analytical Techniques
The development of analytical methods for detecting BDCPP and its metabolites is crucial for advancing research in toxicology and environmental science. A high-throughput method employing isotope dilution mass spectrometry has been established for quantifying multiple organophosphates in urine samples. This method demonstrated high recovery rates (90-113%) and low detection limits, making it suitable for large-scale epidemiological studies .
Data Table: Method Performance
Method Type | Detection Limit (pg/mL) | Recovery Rate (%) | Sample Throughput (samples/day) |
---|---|---|---|
Solid-Phase Extraction + LC-MS/MS | 8 | 90-113 | 96 |
Mixed-mode Anion Exchange + LC-MS/MS | 8 | 82-91 | Variable |
作用機序
The mechanism of action of Bis(1,3-dichloro-2-propyl) Phosphate-d10 involves its interaction with biological molecules. The compound can:
Inhibit Enzymes: It may inhibit enzymes such as acetylcholinesterase, leading to the accumulation of acetylcholine and subsequent neurotoxic effects.
Induce Oxidative Stress: The compound can generate reactive oxygen species (ROS), leading to oxidative stress and cellular damage.
Disrupt Cellular Functions: It can interfere with cellular signaling pathways, affecting various physiological processes.
類似化合物との比較
Chemical Structure and Metabolic Origins
BDCPP-d10 and its analogs share structural similarities but differ in substituents and metabolic pathways:
Compound | Parent Compound | Structure | Key Metabolic Pathway |
---|---|---|---|
BDCPP-d10 | TDCPP (deuterated analog) | Bis(1,3-dichloro-2-propyl) phosphate-d10 | Isotopic labeling for quantification |
BDCPP | TDCPP | Bis(1,3-dichloro-2-propyl) phosphate | Oxidative dealkylation |
Diphenyl phosphate (DPHP) | Triphenyl phosphate (TPHP) | Two phenyl groups attached to phosphate | Hydrolysis of phenyl groups |
BCIPP | TCIPP (tris(1-chloro-2-propyl) phosphate) | Bis(1-chloro-2-propyl) phosphate | Dechlorination and oxidation |
BCEP | TCEP (tris(2-chloroethyl) phosphate) | Bis(2-chloroethyl) phosphate | Dealkylation |
Key Differences :
- Chlorination Pattern : BDCPP and BCIPP contain chlorine atoms on propyl chains, whereas BCEP has chloroethyl groups. DPHP lacks chlorine, making it less persistent in environmental matrices .
- Metabolic Rates : BDCPP is rapidly formed from TDCPP in rodents and humans, with urinary detection within hours of exposure . In contrast, DPHP shows slower excretion kinetics due to TPHP’s higher lipophilicity .
Analytical Detection and Recovery
BDCPP-d10 is critical for accurate quantification of BDCPP in urine and environmental samples. However, extraction efficiency varies among metabolites:
Table 1. Solid-Phase Extraction (SPE) Recovery Rates for BDCPP and Analogs
Compound | Anion Exchange SPE Recovery (%) |
---|---|
BDCPP | 46–83% (varies by SPE cartridge) |
DPHP | <20% (poor retention on anion exchangers) |
Implications :
- BDCPP’s moderate recovery necessitates method optimization, while DPHP’s poor recovery requires alternative approaches like mixed-mode SPE .
- BCIPP and BCEP exhibit intermediate recovery rates (~30–60%), influenced by their polarity and chlorine content .
Table 2. Urinary Concentrations of BDCPP vs. DPHP in Human Studies
Metric (pg/mL) | BDCPP | DPHP |
---|---|---|
Geometric Mean | 148 | 1,074 |
Maximum | 1,662 | 7,443 |
DPHP levels are consistently higher due to TPHP’s widespread use in nail polish and plastics . BDCPP levels reflect TDCPP exposure from furniture and baby products .
Environmental Presence and Persistence
Table 3. Global Detection in Environmental Matrices
Compound | Detection in Fishmeal (%) | Dust (ng/g) | Water (ng/L) |
---|---|---|---|
BDCPP | 100% | 50–500 | 5–20 |
BCEP | 100% | 100–1,000 | 10–50 |
DPHP | 90% | 200–2,000 | 20–100 |
Trends :
- BDCPP and BCEP are ubiquitous in marine ecosystems due to TDCPP and TCEP’s resistance to hydrolysis .
- DPHP dominates in indoor dust due to TPHP’s volatility and use in consumer products .
Toxicity and Health Impacts
Table 4. Comparative Toxicity Endpoints
Compound | Key Health Effects | Mechanism |
---|---|---|
BDCPP | Fetal growth impairment, lung dysfunction | Endocrine disruption, oxidative stress |
BCEP | Renal toxicity, airway remodeling | ROS generation, apoptosis |
DPHP | Adiposity, hormonal imbalance | PPARγ activation |
BCIPP | Developmental neurotoxicity (suspected) | Cholinergic enzyme inhibition |
Notable Findings:
- BDCPP exposure in pregnant women correlates with reduced fetal growth, likely via placental transfer .
- BCEP and BDCPP synergistically impair lung function by activating NF-κB and MAPK pathways .
- DPHP is linked to obesity in adults, with a 1.5-fold increase in adiposity per log-unit increase in urinary DPHP .
生物活性
Bis(1,3-dichloro-2-propyl) phosphate-d10 (BDCPP-d10) is a deuterated form of bis(1,3-dichloro-2-propyl) phosphate, a compound that has gained attention due to its use as a flame retardant and its potential biological impacts. This article reviews the biological activity of BDCPP-d10, focusing on its toxicity, metabolic pathways, and implications for human health.
BDCPP-d10 is an organophosphate flame retardant (OPFR) that has been utilized as an alternative to polybrominated diphenyl ethers (PBDEs), which have been banned due to their environmental persistence and toxicity. The compound is primarily used in various consumer products, including textiles and plastics.
Acute and Chronic Toxicity
Research indicates that BDCPP exhibits significant toxicity in various biological systems. A study showed that exposure to tris(1,3-dichloro-2-propyl) phosphate (TDCPP), the parent compound of BDCPP, resulted in adverse effects such as:
- Liver and kidney damage : High doses led to increased organ weights and histopathological changes in rats .
- Carcinogenic potential : Long-term studies demonstrated tumor formation in high-dose groups of male rats .
- Developmental effects : Evidence suggests that OPFRs like BDCPP may disrupt endocrine functions and affect reproductive health .
Metabolic Pathways
BDCPP is metabolized in humans primarily to bis(1,3-dichloro-2-propyl) phosphate. Studies have shown that:
- Detection in urine : BDCPP was found in 91% of urine samples from nonoccupationally exposed adults, indicating widespread exposure .
- Temporal reliability : The concentrations of BDCPP metabolites showed moderate-to-strong reliability over time, suggesting consistent exposure patterns among individuals .
Human Exposure Studies
A notable study examined urinary metabolites of OPFRs among men over three months. The findings highlighted:
- High detection rates of BDCPP and its metabolites in urine samples.
- Concentrations ranged significantly, with BDCPP levels reaching up to 1.66 ng/mL in some individuals .
Animal Studies
In animal models, particularly rats:
- Long-term exposure to TDCPP resulted in significant weight loss and increased mortality rates among high-dose groups.
- Histological examinations revealed hyperplasia in kidney tubules and other organ-specific changes .
Environmental Persistence
BDCPP possesses properties that allow it to persist in the environment. Its logKow values indicate potential for bioaccumulation, raising concerns about long-term ecological impacts . The compound's resistance to degradation further complicates its environmental management.
Summary of Biological Activity
Biological Activity | Findings |
---|---|
Toxicity | Significant liver and kidney damage; carcinogenic potential observed in animal studies. |
Metabolism | Detected in urine; primary metabolites identified as BDCPP and DPP. |
Environmental Impact | Persistent and bioaccumulative; raises concerns for human health and ecosystems. |
Q & A
Basic Research Questions
Q. What are the critical considerations for synthesizing Bis(1,3-dichloro-2-propyl) Phosphate-d10 with isotopic purity for tracer studies?
Methodological Answer: Synthesis should prioritize isotopic labeling efficiency and purity. Use controlled reaction conditions (e.g., inert atmosphere, anhydrous solvents) to minimize deuterium loss. Analytical validation via nuclear magnetic resonance (NMR) and high-resolution mass spectrometry (HRMS) is essential to confirm isotopic integrity. Ensure separation techniques (e.g., column chromatography) are optimized to remove unlabeled byproducts .
Q. How can researchers ensure the stability of this compound during long-term storage in laboratory settings?
Methodological Answer: Store the compound in airtight, light-resistant containers under inert gas (e.g., argon) at temperatures ≤−20°C. Regularly monitor degradation via gas chromatography-mass spectrometry (GC-MS) or liquid chromatography-tandem mass spectrometry (LC-MS/MS). Avoid exposure to moisture and oxidizers, as hydrolysis or oxidation could alter its structure .
Q. What analytical techniques are most reliable for quantifying this compound in environmental matrices?
Methodological Answer: Solid-phase extraction (SPE) coupled with LC-MS/MS is recommended for trace-level detection in complex matrices. Use deuterated internal standards (e.g., Bis(1,3-dichloro-2-propyl) Phosphate-d15) to correct for matrix effects. Validate method sensitivity via limit of detection (LOD) and limit of quantification (LOQ) calculations, ensuring compliance with EPA or OECD guidelines .
Advanced Research Questions
Q. How can factorial design be applied to optimize the degradation pathways of this compound in aquatic systems?
Methodological Answer: Employ a 2<sup>k</sup> factorial design to evaluate variables such as pH, temperature, and UV exposure. Use response surface methodology (RSM) to model interactions between factors and identify optimal degradation conditions. Validate results with kinetic studies (e.g., pseudo-first-order rate constants) and intermediate identification via Fourier-transform ion cyclotron resonance (FT-ICR) MS .
Q. What theoretical frameworks are critical for interpreting contradictory data on the neurotoxic effects of organophosphate flame retardants like this compound?
Methodological Answer: Integrate the "cholinergic hypothesis" (linking acetylcholinesterase inhibition to neurotoxicity) with metabolomic profiling to resolve discrepancies. Use systems biology models to account for interspecies variability (e.g., zebrafish vs. mammalian models). Cross-validate findings with transcriptomic data to identify conserved pathways .
Q. How can researchers reconcile discrepancies in bioaccumulation factors (BAFs) reported for this compound across different trophic levels?
Methodological Answer: Apply probabilistic modeling (e.g., Monte Carlo simulations) to account for variability in lipid content and trophic transfer efficiency. Use stable isotope tracing (δ<sup>13</sup>C or δ<sup>15</sup>N) in mesocosm studies to track compound movement. Compare results with QSAR (quantitative structure-activity relationship) predictions to identify outliers .
Q. Methodological and Theoretical Integration
Q. What steps should be taken to design a mechanistic study on the interaction of this compound with cellular membranes?
Methodological Answer: Combine molecular dynamics (MD) simulations with experimental biophysical techniques (e.g., surface plasmon resonance or fluorescence anisotropy). Use deuterium-labeled lipids to study compound localization via neutron scattering. Validate membrane permeability assays with confocal microscopy .
Q. How can epistemological frameworks guide the selection of models for assessing the environmental fate of this compound?
Methodological Answer: Adopt a "source-to-receptor" paradigm (as outlined in DOE atmospheric chemistry research) to integrate air-surface exchange, heterogeneous chemistry, and scavenging processes. Align model parameters with field data from analogous organophosphates, ensuring theoretical consistency with partition coefficients (e.g., log Kow) .
Q. Data Validation and Reproducibility
Q. What strategies mitigate batch-to-batch variability in synthesized this compound used for interlaboratory studies?
Methodological Answer: Implement stringent quality control (QC) protocols, including <sup>31</sup>P NMR for phosphorus purity and isotope ratio mass spectrometry (IRMS) for deuterium content. Share raw spectral data and calibration curves via open-access repositories (e.g., Zenodo) to enhance reproducibility .
Q. How should researchers address conflicting results in oxidative degradation studies of this compound?
Methodological Answer: Conduct a meta-analysis of reaction conditions (e.g., oxidant concentration, catalyst type) using PRISMA guidelines. Perform replicate experiments under harmonized protocols and employ multivariate statistics (e.g., principal component analysis) to identify critical variables. Cross-reference with high-throughput screening databases (e.g., PubChem) to contextualize findings .
特性
CAS番号 |
1477495-19-4 |
---|---|
分子式 |
C6H11Cl4O4P |
分子量 |
329.985 |
IUPAC名 |
bis(1,3-dichloro-1,1,2,3,3-pentadeuteriopropan-2-yl) hydrogen phosphate |
InChI |
InChI=1S/C6H11Cl4O4P/c7-1-5(2-8)13-15(11,12)14-6(3-9)4-10/h5-6H,1-4H2,(H,11,12)/i1D2,2D2,3D2,4D2,5D,6D |
InChIキー |
NNKRUBFJSSBFSS-MBXGXEIXSA-N |
SMILES |
C(C(CCl)OP(=O)(O)OC(CCl)CCl)Cl |
同義語 |
1,3-Dichloro-2-propanol-d7 Hydrogen Phosphate; |
製品の起源 |
United States |
試験管内研究製品の免責事項と情報
BenchChemで提示されるすべての記事および製品情報は、情報提供を目的としています。BenchChemで購入可能な製品は、生体外研究のために特別に設計されています。生体外研究は、ラテン語の "in glass" に由来し、生物体の外で行われる実験を指します。これらの製品は医薬品または薬として分類されておらず、FDAから任何の医療状態、病気、または疾患の予防、治療、または治癒のために承認されていません。これらの製品を人間または動物に体内に導入する形態は、法律により厳格に禁止されています。これらのガイドラインに従うことは、研究と実験において法的および倫理的な基準の遵守を確実にするために重要です。