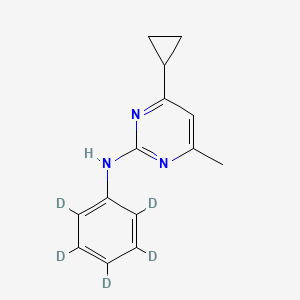
シプロジニル-d5
- 専門家チームからの見積もりを受け取るには、QUICK INQUIRYをクリックしてください。
- 品質商品を競争力のある価格で提供し、研究に集中できます。
説明
Cyprodinil-d5 is an isotope-labeled analog of the pyrimidinamine fungicide cyprodinil, where the phenyl protons are replaced by deuterium. This compound is primarily used in scientific research for the quantitative analysis of pesticides due to its stable isotopic labeling, which allows for precise measurements in mass spectrometry .
科学的研究の応用
Cyprodinil-d5 is extensively used in scientific research, particularly in the following fields:
Chemistry: It is used as an internal standard in isotope dilution mass spectrometry (IDMS) for the accurate quantification of cyprodinil in various samples.
Biology: The compound helps in studying the metabolic pathways of cyprodinil in biological systems.
Medicine: Research involving Cyprodinil-d5 aids in understanding the pharmacokinetics and pharmacodynamics of cyprodinil.
Industry: It is used in environmental analysis to monitor pesticide residues in agricultural products.
作用機序
Target of Action
Cyprodinil-d5, a deuterium-labeled analog of the pyrimidinamine fungicide Cyprodinil , primarily targets the biosynthesis of methionine in phytopathogenic fungi . Methionine is an essential amino acid that plays a crucial role in protein synthesis and various metabolic processes within the fungal cells .
Mode of Action
Cyprodinil-d5 interacts with its target by inhibiting the biosynthesis of methionine . This inhibition disrupts the normal metabolic processes of the fungi, leading to the cessation of their growth and eventual death . It’s worth noting that Cyprodinil-d5 also acts as an androgen receptor (AR) agonist in the absence of the AR agonist DHT and inhibits the androgenic effect of DHT .
Biochemical Pathways
The primary biochemical pathway affected by Cyprodinil-d5 is the methionine biosynthesis pathway in phytopathogenic fungi . By inhibiting this pathway, Cyprodinil-d5 disrupts the production of essential proteins and other metabolites, leading to the inhibition of fungal growth .
Pharmacokinetics
Cyprodinil-d5 is characterized by low solubility and volatility . It is moderately persistent in soils but may be persistent in water systems depending on local conditions . These properties impact its bioavailability and distribution in the environment .
Result of Action
The primary molecular effect of Cyprodinil-d5 is the inhibition of methionine biosynthesis, which leads to the disruption of essential metabolic processes in the fungi . On a cellular level, this results in the inhibition of fungal growth . Additionally, Cyprodinil-d5 has been observed to elicit cardiac abnormality in zebrafish embryos .
Action Environment
Environmental factors can significantly influence the action, efficacy, and stability of Cyprodinil-d5. For instance, its persistence in soils and water systems can be influenced by local conditions . Furthermore, it has been observed that certain naturally occurring compounds, such as Resveratrol, can mitigate the toxic effects of Cyprodinil-d5 .
準備方法
Synthetic Routes and Reaction Conditions: The synthesis of Cyprodinil-d5 involves the deuteration of cyprodinil. The process typically includes the introduction of deuterium atoms into the phenyl ring of cyprodinil. This can be achieved through various chemical reactions that replace hydrogen atoms with deuterium.
Industrial Production Methods: Industrial production of Cyprodinil-d5 follows similar synthetic routes but on a larger scale. The process involves stringent control of reaction conditions to ensure high purity and yield. The compound is then purified using techniques such as high-performance liquid chromatography (HPLC) to achieve the desired isotopic enrichment .
化学反応の分析
Types of Reactions: Cyprodinil-d5 undergoes several types of chemical reactions, including:
Oxidation: The compound can be oxidized under specific conditions to form various oxidized derivatives.
Reduction: Reduction reactions can convert Cyprodinil-d5 into its reduced forms.
Substitution: The deuterium atoms in Cyprodinil-d5 can be substituted with other atoms or groups under appropriate conditions.
Common Reagents and Conditions:
Oxidation: Common oxidizing agents include potassium permanganate and hydrogen peroxide.
Reduction: Reducing agents such as lithium aluminum hydride and sodium borohydride are used.
Substitution: Various nucleophiles can be used for substitution reactions, depending on the desired product.
Major Products: The major products formed from these reactions depend on the specific reagents and conditions used. For example, oxidation may yield hydroxylated derivatives, while reduction can produce deuterated amines .
類似化合物との比較
Cyprodinil: The non-labeled version of Cyprodinil-d5, used widely as a fungicide.
Pyrimethanil: Another anilinopyrimidine fungicide with a similar mode of action.
Fludioxonil: A phenylpyrrole fungicide that also targets fungal growth but through a different mechanism.
Uniqueness: Cyprodinil-d5 is unique due to its isotopic labeling, which provides distinct advantages in analytical applications. The deuterium atoms in Cyprodinil-d5 allow for precise tracking and quantification in mass spectrometry, making it an invaluable tool in scientific research .
生物活性
Cyprodinil-d5 is a stable isotope-labeled derivative of cyprodinil, a broad-spectrum fungicide primarily used in agriculture to control various phytopathogenic fungi. This article explores the biological activity of cyprodinil-d5, focusing on its mechanism of action, effects on enzymatic activity, and implications for environmental and human health.
Cyprodinil is classified as an anilinopyrimidine fungicide. It functions by inhibiting the biosynthesis of methionine in fungi, which is essential for protein synthesis and cellular metabolism. The introduction of deuterium in cyprodinil-d5 allows for enhanced tracking and analysis in biological studies, providing insights into its metabolic pathways and environmental behavior.
Enzymatic Inhibition Studies
A significant aspect of cyprodinil's biological activity is its effect on enzyme systems. A study investigated the impact of cyprodinil on bovine liver catalase (CAT) activity. The results indicated that increasing concentrations of cyprodinil led to a notable decrease in CAT activity, with percentage reductions observed at various concentrations as follows:
Concentration (ppm) | % Decrease in CAT Activity |
---|---|
10 | 45.4 |
50 | 68.0 |
100 | 73.0 |
250 | 77.8 |
500 | 77.4 |
The inhibition was determined to be competitive, suggesting that cyprodinil molecules compete with hydrogen peroxide for binding sites on the CAT enzyme . This competitive inhibition indicates potential oxidative stress implications when organisms are exposed to cyprodinil.
Case Studies and Environmental Impact
Cyprodinil has been studied for its environmental persistence and bioaccumulation potential. Research indicates that while it is effective against target fungi, its residues can accumulate in non-target organisms, raising concerns about ecological toxicity. For instance, studies have shown that exposure to cyprodinil can induce stress responses in aquatic organisms such as mussels, leading to biomarkers indicative of oxidative damage and metabolic disruption .
Research Findings on Toxicological Effects
In addition to its effects on enzymatic activity, cyprodinil has been linked to various toxicological outcomes in non-target species. A comprehensive review highlighted the following findings:
- Oxidative Stress : Exposure to cyprodinil resulted in increased levels of lipid peroxidation and DNA damage in aquatic organisms at concentrations as low as 1 µg/L.
- Bioconcentration Factors : The bioconcentration factor (BCF) for cyprodinil was found to be significant, indicating that it can accumulate in the tissues of aquatic species, potentially leading to higher trophic level impacts .
特性
IUPAC Name |
4-cyclopropyl-6-methyl-N-(2,3,4,5,6-pentadeuteriophenyl)pyrimidin-2-amine |
Source
|
---|---|---|
Source | PubChem | |
URL | https://pubchem.ncbi.nlm.nih.gov | |
Description | Data deposited in or computed by PubChem | |
InChI |
InChI=1S/C14H15N3/c1-10-9-13(11-7-8-11)17-14(15-10)16-12-5-3-2-4-6-12/h2-6,9,11H,7-8H2,1H3,(H,15,16,17)/i2D,3D,4D,5D,6D |
Source
|
Source | PubChem | |
URL | https://pubchem.ncbi.nlm.nih.gov | |
Description | Data deposited in or computed by PubChem | |
InChI Key |
HAORKNGNJCEJBX-VIQYUKPQSA-N |
Source
|
Source | PubChem | |
URL | https://pubchem.ncbi.nlm.nih.gov | |
Description | Data deposited in or computed by PubChem | |
Canonical SMILES |
CC1=CC(=NC(=N1)NC2=CC=CC=C2)C3CC3 |
Source
|
Source | PubChem | |
URL | https://pubchem.ncbi.nlm.nih.gov | |
Description | Data deposited in or computed by PubChem | |
Isomeric SMILES |
[2H]C1=C(C(=C(C(=C1[2H])[2H])NC2=NC(=CC(=N2)C3CC3)C)[2H])[2H] |
Source
|
Source | PubChem | |
URL | https://pubchem.ncbi.nlm.nih.gov | |
Description | Data deposited in or computed by PubChem | |
Molecular Formula |
C14H15N3 |
Source
|
Source | PubChem | |
URL | https://pubchem.ncbi.nlm.nih.gov | |
Description | Data deposited in or computed by PubChem | |
Molecular Weight |
230.32 g/mol |
Source
|
Source | PubChem | |
URL | https://pubchem.ncbi.nlm.nih.gov | |
Description | Data deposited in or computed by PubChem | |
試験管内研究製品の免責事項と情報
BenchChemで提示されるすべての記事および製品情報は、情報提供を目的としています。BenchChemで購入可能な製品は、生体外研究のために特別に設計されています。生体外研究は、ラテン語の "in glass" に由来し、生物体の外で行われる実験を指します。これらの製品は医薬品または薬として分類されておらず、FDAから任何の医療状態、病気、または疾患の予防、治療、または治癒のために承認されていません。これらの製品を人間または動物に体内に導入する形態は、法律により厳格に禁止されています。これらのガイドラインに従うことは、研究と実験において法的および倫理的な基準の遵守を確実にするために重要です。