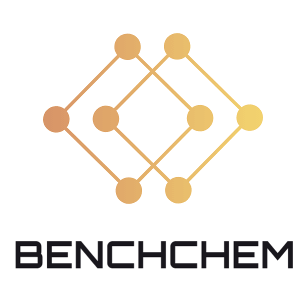
Glycol Chitosan
- 専門家チームからの見積もりを受け取るには、QUICK INQUIRYをクリックしてください。
- 品質商品を競争力のある価格で提供し、研究に集中できます。
説明
Glycol Chitosan (GC) is a derivative of chitosan that has hydrophilic ethylene glycol branches. It is water-soluble, biocompatible, and biodegradable . It has both hydrophobic segments for the encapsulation of various drugs and reactive functional groups for facile chemical modifications .
Synthesis Analysis
GC is a chitosan derivative conjugated with hydrophilic ethylene glycol branches that render the polymer soluble in water at a neutral/acidic pH. The molecular weight of GC ranges from 20 to 250 kDa with the degree of deacetylation from 60 to 82.7% . The reactive functional groups of GC such as amine and hydroxyl groups allow for easy chemical modification with various chemical compounds .Molecular Structure Analysis
The molecular structure of GC includes hydrophilic ethylene glycol branches that render the polymer soluble in water at a neutral/acidic pH. The molecular weight of GC ranges from 20 to 250 kDa with the degree of deacetylation from 60 to 82.7% .Chemical Reactions Analysis
GC and its derivatives have been extensively investigated as safe and effective drug delivery carriers because of their unique physiochemical and biological properties. The reactive functional groups such as the amine and hydroxyl groups on the GC backbone allow for easy chemical modification with various chemical compounds .Physical And Chemical Properties Analysis
GC is a chitosan derivative conjugated with hydrophilic ethylene glycol branches that render the polymer soluble in water at a neutral/acidic pH. The molecular weight of GC ranges from 20 to 250 kDa with the degree of deacetylation from 60 to 82.7% . The reactive functional groups of GC such as amine and hydroxyl groups allow for easy chemical modification with various chemical compounds .科学的研究の応用
Drug Delivery Systems
Glycol Chitosan (GC) and its derivatives are extensively investigated as safe and effective drug delivery carriers due to their unique physicochemical and biological properties. They have hydrophobic segments for encapsulating various drugs and reactive functional groups for facile chemical modifications, making them suitable for targeted drug delivery .
Water Purification
Chitosan-based hydrogels, including those derived from Glycol Chitosan, are noted for their potential in water treatment and purification. Their bioavailability, biocompatibility, biodegradability, environmental friendliness, high pollutants adsorption capacity, and water adsorption capacity make them excellent materials for removing contaminants from water .
Antibacterial Applications
In antibacterial applications, chitosan exhibits potent antimicrobial properties by disrupting microbial membranes and DNA. This makes Glycol Chitosan a promising natural preservative and agent against bacterial infections .
Non-cell Adhesive Material
Glycol Chitosan has been used as an ultralow cell adhesive material. Surfaces coated with chitosan inhibit the attachment of cells and facilitate spheroid formation, which is crucial in tissue engineering and regenerative medicine .
Biomedical Applications
Chitosan and its derivatives, including Glycol Chitosan, have practical applications in various biomedical fields such as pharmacy, medicine, biomedicine, dentistry, ophthalmology, cosmetology, hygiene, personal care, bio-imaging, and veterinary medicine due to their biocompatibility and non-toxicity .
Nanotechnology
Due to its ability to form hydrogels and its reactive functional groups, Glycol Chitosan is also used in nanotechnology applications. It can be used to create nanoparticles for drug delivery or as a component in the synthesis of nanocomposites with enhanced properties .
作用機序
Target of Action
Glycol Chitosan (GC) is a water-soluble chitosan derivative with hydrophilic ethylene glycol branches . It primarily targets pathogenic microorganisms, preventing their growth, sporulation, spore viability, and germination . It also targets cells for drug delivery . The polycationic charge of chitosan is thought to be the most significant factor bringing about antimicrobial effects due to electrostatic interaction between the polycationic chitosan and microbial cell surface .
Mode of Action
GC interacts with its targets through various mechanisms. For pathogenic microorganisms, it disrupts cell function and induces different defense responses in the host plant . In drug delivery, GC encapsulates various drugs within its hydrophobic segments and delivers them to target cells . The presence of hydrophilic ethylene glycol branches and reactive functional groups on the GC backbone allows for facile chemical modifications .
Biochemical Pathways
GC affects several biochemical pathways. It has been shown to synthesize chondrogenic markers (COL2A1 and ACAN), suggesting potential for tissue regeneration . Additionally, GC has been used to synthesize hydrogels that have shown potential in facilitating human biological processes like inflammation .
Pharmacokinetics
The pharmacokinetics of GC-based drug carriers have been studied. These carriers sufficiently accumulate in diseased tissues where there is leaky vasculature and poor lymphatic drainage . This passive tumor-homing based on enhanced permeability and retention (EPR) effects enhances the therapeutic efficacies of drugs while reducing their side effects .
Result of Action
The action of GC results in several molecular and cellular effects. It has been shown to effectively inhibit bacterial growth . In drug delivery, GC enhances the water solubility of sparingly soluble drugs , and in tissue engineering, it has been shown to enhance the synthesis and accumulation of collagen I, II, and aggrecan markers in encapsulated chondrocytes .
Action Environment
The action of GC can be influenced by environmental factors. For instance, the presence of ions can create a large osmotic swelling force that makes the hydrogel swell at a pH below the polymer pKa . Additionally, the solution must be sufficiently acidic to favor the reaction, resulting in protonation of the carbonyl compound and making the carbon more susceptible to nucleophilic attack .
Safety and Hazards
将来の方向性
GC and its derivatives have been extensively investigated as safe and effective drug delivery carriers because of their unique physiochemical and biological properties . This review summarizes the recent advances of GC-based materials in cell surface labeling, multimodal tumor imaging, and encapsulation and delivery of drugs . Different strategies for GC modifications are also highlighted with the aim to shed light on how to endow GC and its derivatives with desirable properties for therapeutic purposes .
特性
{ "Design of the Synthesis Pathway": "Glycol Chitosan can be synthesized by the reaction between chitosan and ethylene oxide.", "Starting Materials": [ "Chitosan", "Ethylene oxide" ], "Reaction": [ "Chitosan is dissolved in acetic acid to form a solution.", "Ethylene oxide is added dropwise to the chitosan solution.", "The reaction mixture is stirred at room temperature for several hours.", "The resulting Glycol Chitosan product is then purified by dialysis and dried." ] } | |
CAS番号 |
123938-86-3 |
分子式 |
C24H47N3O16 |
分子量 |
633.645 |
IUPAC名 |
(3S,4S,6S)-5-amino-6-[(3R,4S,6S)-5-amino-6-[(3R,4S,6R)-5-amino-4,6-dihydroxy-2-(2-hydroxyethoxymethyl)oxan-3-yl]oxy-4-hydroxy-2-(2-hydroxyethoxymethyl)oxan-3-yl]oxy-2-(2-hydroxyethoxymethyl)oxane-3,4-diol |
InChI |
InChI=1S/C24H47N3O16/c25-13-18(33)20(11(39-22(13)35)8-37-5-2-29)42-24-15(27)19(34)21(12(41-24)9-38-6-3-30)43-23-14(26)17(32)16(31)10(40-23)7-36-4-1-28/h10-24,28-35H,1-9,25-27H2/t10?,11?,12?,13?,14?,15?,16-,17+,18+,19+,20+,21+,22-,23+,24+/m1/s1 |
InChIキー |
RKEUQJJGKWLRQI-PGHSOFRXSA-N |
SMILES |
C(COCC1C(C(C(C(O1)OC2C(OC(C(C2O)N)OC3C(OC(C(C3O)N)O)COCCO)COCCO)N)O)O)O |
同義語 |
100D-VL; Amidan; Armour-Zen; BC 10; BC 10 (polysaccharide); Batch LO 7319CsU; Biochemica; Biochikol; Biochikol 020PC; Biopolymer L 112; C 3646; C 60M; CG 10; CG 110; CTA 1 Lactic Acid; CTA 4; Cerosan 5000; ChiSys; Chicol; Chiloclear; Chimarin; Chiros |
製品の起源 |
United States |
試験管内研究製品の免責事項と情報
BenchChemで提示されるすべての記事および製品情報は、情報提供を目的としています。BenchChemで購入可能な製品は、生体外研究のために特別に設計されています。生体外研究は、ラテン語の "in glass" に由来し、生物体の外で行われる実験を指します。これらの製品は医薬品または薬として分類されておらず、FDAから任何の医療状態、病気、または疾患の予防、治療、または治癒のために承認されていません。これらの製品を人間または動物に体内に導入する形態は、法律により厳格に禁止されています。これらのガイドラインに従うことは、研究と実験において法的および倫理的な基準の遵守を確実にするために重要です。