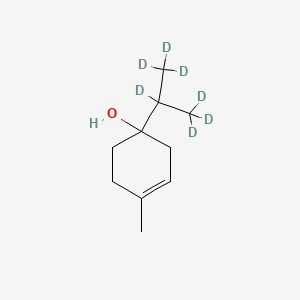
ラセミ体 テルピネン-4-オール-d7
- 専門家チームからの見積もりを受け取るには、QUICK INQUIRYをクリックしてください。
- 品質商品を競争力のある価格で提供し、研究に集中できます。
説明
rac Terpinen-4-ol-d7 is a deuterated form of Terpinen-4-ol, a naturally occurring monoterpene alcohol. It is primarily known for its antiseptic and antioxidant properties . The compound has a molecular formula of C10H11D7O and a molecular weight of 161.29 . The deuterium labeling makes it particularly useful in scientific research, especially in studies involving mass spectrometry.
科学的研究の応用
rac Terpinen-4-ol-d7 is widely used in scientific research due to its unique properties:
Biology: The compound is used in studies involving metabolic pathways and enzyme kinetics due to its stable isotope labeling.
Industry: Used in the formulation of various industrial products, including coatings and adhesives, due to its chemical stability and reactivity.
作用機序
Target of Action
rac Terpinen-4-ol-d7, also known as Terpinen-4-ol-d7, is primarily known for its antiseptic properties . It has been shown to have significant effects on macrophages , which are key cells in the immune system that participate in all stages of immune responses .
Mode of Action
Terpinen-4-ol-d7 interacts with its targets, primarily macrophages, by inducing a significant decrease in the expression levels of inflammatory cytokines induced by lipopolysaccharide (LPS) . It also shows antioxidant effects .
Biochemical Pathways
Terpinen-4-ol-d7 affects several biochemical pathways. It mainly impacts glutamine and glutamate metabolism and glycine, serine, and threonine metabolic pathways . It increases the transcript levels of GLS and GDH, promoting glutamine catabolism .
Result of Action
The overall results of Terpinen-4-ol-d7’s action include the inhibition of mTOR phosphorylation to promote glutamine metabolism and increased cell oxidative phosphorylation levels . This leads to the inhibition of the expression of LPS-induced inflammatory cytokines . It also demonstrates a dose-dependent inhibition of the growth of colorectal, pancreatic, prostate, and gastric cancer cells .
生化学分析
Biochemical Properties
Rac Terpinen-4-ol-d7 is known to interact with various enzymes and proteins. For instance, it has been found to downregulate Rho-associated coiled-coil containing protein kinase 2 (ROCK2), a key target in pancreatic cancer cells . This interaction significantly reduces the expression of ROCK2, thereby inhibiting the proliferation and mobility of pancreatic cancer cells .
Cellular Effects
Rac Terpinen-4-ol-d7 has significant effects on various types of cells and cellular processes. In pancreatic cancer cells, it suppresses cell proliferation and colony formation, induces apoptosis, and inhibits migration and invasion . In addition, it has been found to cause a dose- and time-dependent decrease in the cell survival of IHMGECs .
Molecular Mechanism
The molecular mechanism of action of Rac Terpinen-4-ol-d7 involves binding interactions with biomolecules and changes in gene expression. It exerts its effects at the molecular level by inhibiting the activation of the NF-κB and NLRP3 inflammasome . It also reduces the expression of ROCK2, thereby suppressing the malignant biological behavior of pancreatic cancer cells .
Temporal Effects in Laboratory Settings
Rac Terpinen-4-ol-d7 shows changes in its effects over time in laboratory settings. For instance, it has been found to strongly inhibit DNA and RNA biosynthesis in MRSA at 2 hours after treatment by affecting genes and metabolites related to purine and pyrimidine metabolic pathways .
Dosage Effects in Animal Models
The effects of Rac Terpinen-4-ol-d7 vary with different dosages in animal models. For example, it has been found to alleviate disease activity index (DAI), colon length shortening, colonic pathological damage, and myeloperoxidase (MPO) activities in a dextran sulfate sodium (DSS)-induced experimental colitis model in mice .
Metabolic Pathways
Rac Terpinen-4-ol-d7 is involved in various metabolic pathways. It has been found to affect glutamine and glutamate metabolism and glycine, serine, and threonine metabolic pathways .
準備方法
Synthetic Routes and Reaction Conditions: The synthesis of rac Terpinen-4-ol-d7 typically involves the deuteration of Terpinen-4-ol. This can be achieved through catalytic hydrogenation using deuterium gas (D2) in the presence of a suitable catalyst such as palladium on carbon (Pd/C). The reaction is carried out under controlled temperature and pressure conditions to ensure complete deuteration of the hydrogen atoms in the molecule.
Industrial Production Methods: Industrial production of rac Terpinen-4-ol-d7 follows similar synthetic routes but on a larger scale. The process involves the use of high-pressure reactors and continuous flow systems to maintain the reaction conditions. The product is then purified using techniques such as distillation and chromatography to achieve the desired purity levels.
化学反応の分析
Types of Reactions: rac Terpinen-4-ol-d7 undergoes various chemical reactions, including:
Oxidation: It can be oxidized to form Terpinen-4-one using oxidizing agents such as potassium permanganate (KMnO4) or chromium trioxide (CrO3).
Reduction: The compound can be reduced to form Terpinen-4-ol using reducing agents like lithium aluminum hydride (LiAlH4).
Substitution: It can undergo nucleophilic substitution reactions where the hydroxyl group is replaced by other functional groups.
Common Reagents and Conditions:
Oxidation: Potassium permanganate in an acidic medium.
Reduction: Lithium aluminum hydride in anhydrous ether.
Substitution: Various nucleophiles under basic or acidic conditions.
Major Products Formed:
Oxidation: Terpinen-4-one.
Reduction: Terpinen-4-ol.
Substitution: Depending on the nucleophile, various substituted derivatives of Terpinen-4-ol-d7.
類似化合物との比較
Terpinen-4-ol: The non-deuterated form, widely used for its antiseptic properties.
α-Terpineol: Another monoterpene alcohol with similar antimicrobial properties.
1,8-Cineole: A monoterpene ether with comparable biological activities.
Uniqueness of rac Terpinen-4-ol-d7: The deuterium labeling in rac Terpinen-4-ol-d7 provides enhanced stability and allows for precise tracking in metabolic studies. This makes it particularly valuable in research applications where isotopic labeling is required.
特性
IUPAC Name |
1-(1,1,1,2,3,3,3-heptadeuteriopropan-2-yl)-4-methylcyclohex-3-en-1-ol |
Source
|
---|---|---|
Source | PubChem | |
URL | https://pubchem.ncbi.nlm.nih.gov | |
Description | Data deposited in or computed by PubChem | |
InChI |
InChI=1S/C10H18O/c1-8(2)10(11)6-4-9(3)5-7-10/h4,8,11H,5-7H2,1-3H3/i1D3,2D3,8D |
Source
|
Source | PubChem | |
URL | https://pubchem.ncbi.nlm.nih.gov | |
Description | Data deposited in or computed by PubChem | |
InChI Key |
WRYLYDPHFGVWKC-UNAVHCQLSA-N |
Source
|
Source | PubChem | |
URL | https://pubchem.ncbi.nlm.nih.gov | |
Description | Data deposited in or computed by PubChem | |
Canonical SMILES |
CC1=CCC(CC1)(C(C)C)O |
Source
|
Source | PubChem | |
URL | https://pubchem.ncbi.nlm.nih.gov | |
Description | Data deposited in or computed by PubChem | |
Isomeric SMILES |
[2H]C([2H])([2H])C([2H])(C1(CCC(=CC1)C)O)C([2H])([2H])[2H] |
Source
|
Source | PubChem | |
URL | https://pubchem.ncbi.nlm.nih.gov | |
Description | Data deposited in or computed by PubChem | |
Molecular Formula |
C10H18O |
Source
|
Source | PubChem | |
URL | https://pubchem.ncbi.nlm.nih.gov | |
Description | Data deposited in or computed by PubChem | |
Molecular Weight |
161.29 g/mol |
Source
|
Source | PubChem | |
URL | https://pubchem.ncbi.nlm.nih.gov | |
Description | Data deposited in or computed by PubChem | |
試験管内研究製品の免責事項と情報
BenchChemで提示されるすべての記事および製品情報は、情報提供を目的としています。BenchChemで購入可能な製品は、生体外研究のために特別に設計されています。生体外研究は、ラテン語の "in glass" に由来し、生物体の外で行われる実験を指します。これらの製品は医薬品または薬として分類されておらず、FDAから任何の医療状態、病気、または疾患の予防、治療、または治癒のために承認されていません。これらの製品を人間または動物に体内に導入する形態は、法律により厳格に禁止されています。これらのガイドラインに従うことは、研究と実験において法的および倫理的な基準の遵守を確実にするために重要です。