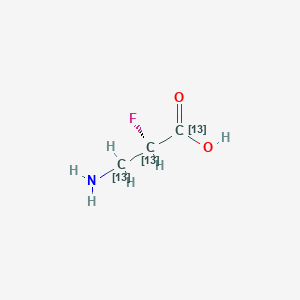
(R)-3-amino-2-fluoropropanoic-1,2,3-13C3 acid
- 専門家チームからの見積もりを受け取るには、QUICK INQUIRYをクリックしてください。
- 品質商品を競争力のある価格で提供し、研究に集中できます。
説明
(R)-3-amino-2-fluoropropanoic-1,2,3-13C3 acid is a fluorinated amino acid derivative. This compound is characterized by the presence of a fluorine atom at the second carbon position and an amino group at the third carbon position. The “13C3” notation indicates that the compound contains three carbon atoms labeled with the carbon-13 isotope, making it useful for various research applications, particularly in nuclear magnetic resonance (NMR) spectroscopy.
準備方法
Synthetic Routes and Reaction Conditions: The synthesis of (R)-3-amino-2-fluoropropanoic-1,2,3-13C3 acid typically involves the introduction of a fluorine atom into a suitable precursor molecule. One common method is the fluorination of a protected amino acid derivative using a fluorinating agent such as diethylaminosulfur trifluoride (DAST) under controlled conditions. The reaction is usually carried out in an inert atmosphere to prevent unwanted side reactions.
Industrial Production Methods: Industrial production of this compound may involve large-scale fluorination processes using automated reactors. The use of isotopically labeled precursors ensures the incorporation of carbon-13 atoms. The final product is purified using techniques such as crystallization or chromatography to achieve the desired purity and isotopic enrichment.
化学反応の分析
Types of Reactions: (R)-3-amino-2-fluoropropanoic-1,2,3-13C3 acid can undergo various chemical reactions, including:
Oxidation: The amino group can be oxidized to form corresponding imines or nitriles.
Reduction: The carboxyl group can be reduced to form alcohols or aldehydes.
Substitution: The fluorine atom can be substituted with other nucleophiles under appropriate conditions.
Common Reagents and Conditions:
Oxidation: Reagents such as potassium permanganate (KMnO4) or hydrogen peroxide (H2O2) can be used.
Reduction: Reagents like lithium aluminum hydride (LiAlH4) or sodium borohydride (NaBH4) are commonly employed.
Substitution: Nucleophiles such as amines or thiols can be used in the presence of catalysts like palladium.
Major Products: The major products formed from these reactions depend on the specific conditions and reagents used. For example, oxidation of the amino group may yield nitriles, while reduction of the carboxyl group may produce alcohols.
科学的研究の応用
(R)-3-amino-2-fluoropropanoic-1,2,3-13C3 acid has a wide range of applications in scientific research:
Chemistry: It is used as a building block in the synthesis of complex molecules and as a probe in NMR spectroscopy due to its isotopic labeling.
Biology: The compound can be incorporated into peptides and proteins to study their structure and function.
Medicine: It is used in the development of fluorinated pharmaceuticals and as a tracer in metabolic studies.
Industry: The compound is employed in the production of specialty chemicals and materials with unique properties.
作用機序
The mechanism of action of (R)-3-amino-2-fluoropropanoic-1,2,3-13C3 acid involves its interaction with specific molecular targets. The fluorine atom can influence the compound’s electronic properties, affecting its binding affinity and reactivity. In biological systems, the compound may interact with enzymes and receptors, altering their activity and function. The carbon-13 labeling allows for detailed studies of these interactions using NMR spectroscopy.
類似化合物との比較
3-Amino-2-fluoropropanoic Acid: Lacks the carbon-13 labeling.
2-Fluoroalanine: Similar structure but different stereochemistry.
Fluorinated Amino Acids: A broad class of compounds with varying positions of fluorine substitution.
Uniqueness: (R)-3-amino-2-fluoropropanoic-1,2,3-13C3 acid is unique due to its specific stereochemistry, fluorine substitution, and isotopic labeling. These features make it particularly valuable for research applications that require precise structural and functional analysis.
生物活性
(R)-3-amino-2-fluoropropanoic acid, particularly in its isotopically labeled form (1,2,3-^13C_3), is a derivative of the amino acid alanine and plays a significant role in various biochemical pathways. This compound is notably recognized for its involvement in the catabolism of the anticancer drug 5-fluorouracil (5-FU). Understanding its biological activity is crucial for evaluating its potential applications in cancer research and other therapeutic areas.
- Molecular Formula : 13C3H6FNO2
- Molecular Weight : 110.06 g/mol
- CAS Number : 1217608-72-4
Biological Activity Overview
(R)-3-amino-2-fluoropropanoic acid acts primarily as a metabolite of 5-fluorouracil, a chemotherapeutic agent used in cancer treatment. The biological activity of this compound can be summarized as follows:
- Metabolic Pathway : It is involved in the metabolic degradation of 5-FU, contributing to the formation of various metabolites that can exert therapeutic effects or toxicity.
- Anticancer Mechanism : The compound's role in the metabolism of 5-FU suggests it may influence the efficacy and toxicity profiles of this drug, which is critical for optimizing cancer treatment regimens.
1. Catabolism of 5-Fluorouracil
The catabolism of 5-FU leads to the formation of several metabolites, including (R)-3-amino-2-fluoropropanoic acid. A study by Gani et al. (1985) elucidated the stereochemistry involved in this process, highlighting how the metabolic pathways can affect drug efficacy and safety profiles .
2. Toxicity Studies
Research has indicated that metabolites such as alpha-fluoro-beta-alanine (FBAL), derived from 5-FU, exhibit neurotoxic effects. These findings underscore the importance of understanding how (R)-3-amino-2-fluoropropanoic acid and its derivatives contribute to both therapeutic outcomes and potential side effects .
3. Analytical Methods
The detection and quantification of (R)-3-amino-2-fluoropropanoic acid in biological samples have been facilitated by advanced analytical techniques such as HPLC-MS/MS. These methods enable researchers to monitor exposure levels in clinical settings, particularly among healthcare workers handling 5-FU .
Case Studies
Several case studies have explored the implications of (R)-3-amino-2-fluoropropanoic acid in clinical settings:
- Case Study on Drug Monitoring : A study focused on urinary biomarkers for monitoring occupational exposure to 5-FU demonstrated that measuring levels of (R)-3-amino-2-fluoropropanoic acid could provide insights into patient exposure and safety .
- Neurotoxicity Assessment : Another case examined the neurotoxic effects associated with FBAL and related compounds, emphasizing the need for careful monitoring of metabolites like (R)-3-amino-2-fluoropropanoic acid during cancer therapy .
Data Table: Summary of Biological Activities
Activity | Description | Reference |
---|---|---|
Metabolic Role | Catabolite of 5-Fluorouracil | Gani et al., 1985 |
Neurotoxicity | Potential neurotoxic effects via FBAL | Journal of Toxicology |
Analytical Monitoring | Detected using HPLC-MS/MS for occupational exposure | ResearchGate |
特性
IUPAC Name |
(2S)-3-amino-2-fluoro(1,2,3-13C3)propanoic acid |
Source
|
---|---|---|
Source | PubChem | |
URL | https://pubchem.ncbi.nlm.nih.gov | |
Description | Data deposited in or computed by PubChem | |
InChI |
InChI=1S/C3H6FNO2/c4-2(1-5)3(6)7/h2H,1,5H2,(H,6,7)/t2-/m0/s1/i1+1,2+1,3+1 |
Source
|
Source | PubChem | |
URL | https://pubchem.ncbi.nlm.nih.gov | |
Description | Data deposited in or computed by PubChem | |
InChI Key |
OJQNRNQELNLWHH-GCCOVPGMSA-N |
Source
|
Source | PubChem | |
URL | https://pubchem.ncbi.nlm.nih.gov | |
Description | Data deposited in or computed by PubChem | |
Canonical SMILES |
C(C(C(=O)O)F)N |
Source
|
Source | PubChem | |
URL | https://pubchem.ncbi.nlm.nih.gov | |
Description | Data deposited in or computed by PubChem | |
Isomeric SMILES |
[13CH2]([13C@@H]([13C](=O)O)F)N |
Source
|
Source | PubChem | |
URL | https://pubchem.ncbi.nlm.nih.gov | |
Description | Data deposited in or computed by PubChem | |
Molecular Formula |
C3H6FNO2 |
Source
|
Source | PubChem | |
URL | https://pubchem.ncbi.nlm.nih.gov | |
Description | Data deposited in or computed by PubChem | |
Molecular Weight |
110.062 g/mol |
Source
|
Source | PubChem | |
URL | https://pubchem.ncbi.nlm.nih.gov | |
Description | Data deposited in or computed by PubChem | |
試験管内研究製品の免責事項と情報
BenchChemで提示されるすべての記事および製品情報は、情報提供を目的としています。BenchChemで購入可能な製品は、生体外研究のために特別に設計されています。生体外研究は、ラテン語の "in glass" に由来し、生物体の外で行われる実験を指します。これらの製品は医薬品または薬として分類されておらず、FDAから任何の医療状態、病気、または疾患の予防、治療、または治癒のために承認されていません。これらの製品を人間または動物に体内に導入する形態は、法律により厳格に禁止されています。これらのガイドラインに従うことは、研究と実験において法的および倫理的な基準の遵守を確実にするために重要です。