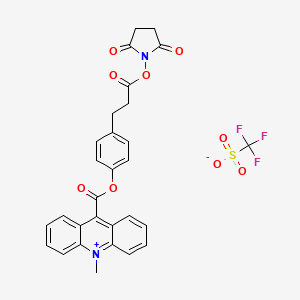
Acridinium C2 NHS Ester
- 専門家チームからの見積もりを受け取るには、QUICK INQUIRYをクリックしてください。
- 品質商品を競争力のある価格で提供し、研究に集中できます。
説明
Acridinium C2 NHS Ester is a chemiluminescent compound widely used in biochemical and medical research. It is known for its ability to label proteins and nucleic acids, generating light in the presence of hydrogen peroxide. This property makes it a valuable tool in various detection methods, including immunoassays and other biological detections .
作用機序
Target of Action
The primary targets of Acridinium C2 NHS Ester are proteins and nucleic acids . The compound is amine-reactive and will bind to any primary amino group on proteins/peptides .
Mode of Action
This compound interacts with its targets (proteins and nucleic acids) through a covalent bond . The compound has a quaternary nitrogen, which promotes a rapid chemiluminescent reaction with hydrogen peroxide . The acridine unit gives rise to the excited state N-methylacridone luminescent emitter . An aryloxy-leaving group is expelled during the reaction with hydrogen peroxide to give rise to a key intermediate in the chemiluminescent reaction .
Biochemical Pathways
The biochemical pathway primarily affected by this compound is the chemiluminescent reaction with hydrogen peroxide . The compound generates chemiluminescence in the presence of hydrogen peroxide . Exposure of an acridinium ester label to an alkaline hydrogen peroxide solution triggers a flash of light .
Pharmacokinetics
The pharmacokinetics of this compound primarily involve its interaction with hydrogen peroxide. The acridinium label is released from the carrier (such as proteins) upon hydrogen peroxide activation . This suggests that the compound’s bioavailability may be influenced by the presence and concentration of hydrogen peroxide in the environment.
Result of Action
The result of this compound’s action is the generation of chemiluminescence. The covalently bound acridinium NHS esters generate chemiluminescence in the presence of hydrogen peroxide . This chemiluminescence can be used as a sensitive detection method in immunoassays and other biological detections .
Action Environment
The action of this compound is influenced by environmental factors such as the presence and concentration of hydrogen peroxide . The compound’s efficacy and stability may also be affected by the pH of the environment, as the chemiluminescent reaction is triggered by exposure to an alkaline hydrogen peroxide solution .
生化学分析
Biochemical Properties
The covalently bound acridinium NHS esters generate chemiluminescence in the presence of hydrogen peroxide . This property makes it a valuable tool in immunoassays and other biological detections .
Cellular Effects
The primary cellular effect of Acridinium C2 NHS Ester is the generation of chemiluminescence in the presence of hydrogen peroxide . This chemiluminescence can be detected and measured, providing a sensitive method for tracking the labeled biomolecules within the cell .
Molecular Mechanism
The molecular mechanism of this compound involves a reaction with hydrogen peroxide . Exposure of an acridinium ester label to an alkaline hydrogen peroxide solution triggers a flash of light . This light emission is the result of a chemical reaction that forms a highly strained dioxetan structure, which breaks down with the generation of an excited state acridone .
Temporal Effects in Laboratory Settings
The effects of this compound can be observed over time in laboratory settings. The chemiluminescence generated by the compound can be detected and measured, providing a temporal record of the labeled biomolecules
Metabolic Pathways
Its primary reaction involves hydrogen peroxide, suggesting that it may interact with metabolic pathways involving this molecule .
準備方法
Synthetic Routes and Reaction Conditions
The synthesis of Acridinium C2 NHS Ester involves the reaction of acridinium derivatives with N-hydroxysuccinimide (NHS) esters. The process typically includes the following steps:
Formation of Acridinium Derivative: Acridinium derivatives are synthesized by reacting acridine with suitable reagents to introduce functional groups.
Coupling with NHS Ester: The acridinium derivative is then coupled with N-hydroxysuccinimide ester in the presence of a coupling agent like dicyclohexylcarbodiimide (DCC) to form the final product.
Industrial Production Methods
Industrial production of this compound follows similar synthetic routes but on a larger scale. The process is optimized for yield and purity, often involving advanced purification techniques like high-performance liquid chromatography (HPLC) to ensure the quality of the final product .
化学反応の分析
Types of Reactions
Acridinium C2 NHS Ester undergoes several types of chemical reactions, including:
Oxidation: In the presence of hydrogen peroxide, the compound undergoes oxidation, leading to the generation of chemiluminescence.
Substitution: The NHS ester group can react with primary amines, leading to the formation of stable amide bonds.
Common Reagents and Conditions
Oxidation: Hydrogen peroxide in an alkaline solution is commonly used to trigger chemiluminescence.
Substitution: Primary amines in a suitable buffer (e.g., phosphate-buffered saline) are used for labeling proteins and other biomolecules.
Major Products Formed
Chemiluminescence Reaction: The major product is light emission, along with the formation of acridinone and carbon dioxide.
Substitution Reaction: The major product is the labeled biomolecule with a stable amide bond.
科学的研究の応用
Acridinium C2 NHS Ester has a wide range of applications in scientific research:
Chemistry: Used as a chemiluminescent label in various analytical techniques.
Biology: Employed in labeling proteins, nucleic acids, and other biomolecules for detection and quantification.
Medicine: Utilized in diagnostic assays, including immunoassays for detecting specific proteins or pathogens.
Industry: Applied in quality control and testing of biological products.
類似化合物との比較
Acridinium C2 NHS Ester is unique compared to other similar compounds due to its specific chemiluminescent properties and stability. Similar compounds include:
Lumiwox™ Acridinium NHS Ester: Unlike this compound, the chemiluminescent label in Lumiwox™ remains bonded to the carrier molecule upon activation.
Acridinium Dimethylphenyl Ester: This compound has different conjugation sites and chemiluminescent properties.
Conclusion
This compound is a versatile and valuable compound in scientific research, offering unique chemiluminescent properties for labeling and detection applications. Its ability to generate light in the presence of hydrogen peroxide makes it an essential tool in various fields, including chemistry, biology, medicine, and industry.
生物活性
Acridinium C2 NHS Ester (CAS Number: 177332-37-5) is a chemiluminescent compound widely utilized in biochemical and medical research for its ability to label proteins and nucleic acids. This compound generates light in the presence of hydrogen peroxide, making it invaluable in various detection methods, particularly in immunoassays. This article explores the biological activity of this compound, detailing its mechanism of action, applications, and relevant research findings.
Target Interaction
this compound primarily targets proteins and nucleic acids. It forms covalent bonds with these biomolecules through its N-hydroxysuccinimide (NHS) ester group, facilitating the labeling process necessary for subsequent detection methods.
Chemiluminescence Generation
The core biochemical pathway affected by this compound involves a reaction with hydrogen peroxide. Upon exposure to an alkaline hydrogen peroxide solution, the compound undergoes oxidation, leading to the generation of chemiluminescence. The reaction can be summarized as follows:
This light emission serves as a signal for the presence of the labeled biomolecules, allowing for sensitive detection in various assays .
Applications
This compound is employed across multiple fields due to its unique properties:
- Immunoassays : It is extensively used for labeling antibodies and antigens, enhancing the sensitivity and specificity of diagnostic tests.
- Biochemical Research : The compound facilitates the study of protein interactions and nucleic acid dynamics by providing a visual signal upon reaction with target molecules.
- Clinical Diagnostics : Acridinium-labeled probes are integral to detecting specific proteins or pathogens in clinical samples, improving diagnostic accuracy .
Research Findings
Recent studies have highlighted the advancements and applications of this compound:
- Sensitivity Improvements : Research indicates that assays utilizing acridinium esters demonstrate superior sensitivity compared to traditional methods. The flexibility of acridinium structures allows for optimization tailored to specific assay requirements .
- Case Study - Immunoassays : A study evaluating the performance of acridinium-based assays showed that they significantly outperformed non-chemiluminescent methods in terms of detection limits and specificity for various biomarkers .
- Temporal Effects : Experiments have demonstrated that chemiluminescence can be measured over time, providing valuable data on the kinetics of labeled biomolecules in laboratory settings. This temporal aspect is crucial for understanding dynamic biological processes .
Data Table: Properties and Applications
Property | Details |
---|---|
Molecular Weight | 632.56 g/mol |
Solubility | Soluble in DMSO or methanol |
Storage Conditions | Store at -20°C |
Primary Applications | Immunoassays, protein labeling, nucleic acid detection |
Mechanism of Action | Generates light via oxidation with H₂O₂ |
特性
IUPAC Name |
[4-[3-(2,5-dioxopyrrolidin-1-yl)oxy-3-oxopropyl]phenyl] 10-methylacridin-10-ium-9-carboxylate;trifluoromethanesulfonate |
Source
|
---|---|---|
Source | PubChem | |
URL | https://pubchem.ncbi.nlm.nih.gov | |
Description | Data deposited in or computed by PubChem | |
InChI |
InChI=1S/C28H23N2O6.CHF3O3S/c1-29-22-8-4-2-6-20(22)27(21-7-3-5-9-23(21)29)28(34)35-19-13-10-18(11-14-19)12-17-26(33)36-30-24(31)15-16-25(30)32;2-1(3,4)8(5,6)7/h2-11,13-14H,12,15-17H2,1H3;(H,5,6,7)/q+1;/p-1 |
Source
|
Source | PubChem | |
URL | https://pubchem.ncbi.nlm.nih.gov | |
Description | Data deposited in or computed by PubChem | |
InChI Key |
NFDRKKHKYOEOLR-UHFFFAOYSA-M |
Source
|
Source | PubChem | |
URL | https://pubchem.ncbi.nlm.nih.gov | |
Description | Data deposited in or computed by PubChem | |
Canonical SMILES |
C[N+]1=C2C=CC=CC2=C(C3=CC=CC=C31)C(=O)OC4=CC=C(C=C4)CCC(=O)ON5C(=O)CCC5=O.C(F)(F)(F)S(=O)(=O)[O-] |
Source
|
Source | PubChem | |
URL | https://pubchem.ncbi.nlm.nih.gov | |
Description | Data deposited in or computed by PubChem | |
Molecular Formula |
C29H23F3N2O9S |
Source
|
Source | PubChem | |
URL | https://pubchem.ncbi.nlm.nih.gov | |
Description | Data deposited in or computed by PubChem | |
Molecular Weight |
632.6 g/mol |
Source
|
Source | PubChem | |
URL | https://pubchem.ncbi.nlm.nih.gov | |
Description | Data deposited in or computed by PubChem | |
試験管内研究製品の免責事項と情報
BenchChemで提示されるすべての記事および製品情報は、情報提供を目的としています。BenchChemで購入可能な製品は、生体外研究のために特別に設計されています。生体外研究は、ラテン語の "in glass" に由来し、生物体の外で行われる実験を指します。これらの製品は医薬品または薬として分類されておらず、FDAから任何の医療状態、病気、または疾患の予防、治療、または治癒のために承認されていません。これらの製品を人間または動物に体内に導入する形態は、法律により厳格に禁止されています。これらのガイドラインに従うことは、研究と実験において法的および倫理的な基準の遵守を確実にするために重要です。