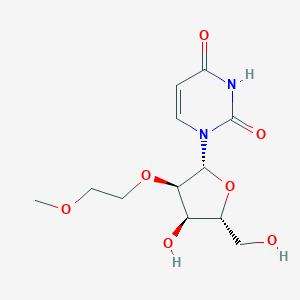
2'-O-(2-Methoxyethyl)-uridine
概要
説明
2'-O-(2-Methoxyethyl)-uridine is a chemically modified nucleoside characterized by a 2'-hydroxyl group substituted with a 2-methoxyethyl (MOE) moiety. This modification enhances the molecule’s resistance to enzymatic degradation and improves its binding affinity to RNA targets, making it critical in therapeutic antisense oligonucleotides (ASOs) . The compound is synthesized via a ring-opening reaction of O-2,2'-anhydro-5-methyluridine with tris-(2-methoxyethyl)borate under optimized conditions, followed by continuous extraction for purification . Its molecular formula is C₁₂H₁₈N₂O₇, with an average mass of 314.28 g/mol .
準備方法
Synthetic Routes and Reaction Conditions: The synthesis of 2’-O-(2-Methoxyethyl)-uridine typically involves the protection of the hydroxyl groups at the 3’ and 5’ positions of uridine. This is followed by the selective alkylation of the 2’-hydroxyl group with 2-methoxyethyl chloride in the presence of a base such as sodium hydride or potassium carbonate. The final step involves the deprotection of the 3’ and 5’ hydroxyl groups to yield the desired product .
Industrial Production Methods: Industrial production of 2’-O-(2-Methoxyethyl)-uridine follows similar synthetic routes but on a larger scale. The process involves optimizing reaction conditions to maximize yield and purity. This includes controlling temperature, reaction time, and the concentration of reagents. The use of continuous flow reactors and automated synthesis platforms can further enhance the efficiency of production .
化学反応の分析
Table 1: Comparison of Ring-Opening Reaction Conditions
Reagent | Catalyst | Reaction Time | Yield (%) | Source |
---|---|---|---|---|
Methanol | Mg | 5 h | 85 | |
2-Methoxyethanol | Al | 48 h | 55 |
Iodination at Position 5
The 5-position of the uracil base undergoes iodination to produce 5-iodo-2'-O-MOE-uridine , a precursor for further functionalization:
- Reagents : N-Iodosuccinimide (NIS) and Ag₂SO₄ in methanol .
- Conditions : Room temperature, 12 hours.
- Yield : 72% for 2'-O-MOE-5-iodo-uridine .
Trifluoromethylation via Radical Mechanism
The 5-position can be modified with a trifluoromethyl group using a radical-based protocol:
- Reagents : Sodium trifluoromethanesulfinate (NaSO₂CF₃) and tert-butylhydroperoxide .
- Conditions : Room temperature, 26 hours.
- Yield : 29% for 2'-O-MOE-5-trifluoromethyl-uridine .
Phosphorylation and Metabolic Stability
2'-O-MOE-uridine exhibits resistance to phosphorylation by nucleotide salvage pathway enzymes, a critical feature for therapeutic safety:
- Enzyme Assays : Deoxycytidine kinase (dCK) and thymidine kinase (TK1) show negligible activity toward 2'-O-MOE-uridine .
- Biological Impact : No incorporation into cellular DNA/RNA observed, unlike 2'-fluoro-modified nucleosides .
Table 2: Phosphorylation Efficiency Comparison
Nucleoside Modification | dCK Activity | TK1 Activity | DNA/RNA Incorporation |
---|---|---|---|
2'-O-MOE-uridine | Negligible | Negligible | None |
2'-F-uridine | High | High | Detected |
Benzoylation Optimization
Benzoylation of intermediates is optimized to minimize side reactions:
- Reagents : Benzoyl chloride with selective hydrolysis of ester impurities .
- Purpose : Protects hydroxyl groups during cytidine analog synthesis .
Comparative Reactivity with Analogs
2'-O-MOE-uridine demonstrates distinct reactivity compared to structurally similar nucleosides:
Table 3: Reactivity Comparison of Uridine Derivatives
Compound | Key Modification | Reactivity Highlights |
---|---|---|
2'-O-Methyluridine | 2'-O-methyl | Lower nuclease resistance than 2'-O-MOE |
2-Thiouridine | 2-thio substitution | Enhanced thermal stability, no alkylation |
5-Methyluridine | 5-methyl | Altered base pairing, no ribose modification |
Stability in Physiological Media
科学的研究の応用
Stability and Resistance
The incorporation of 2'-O-(2-Methoxyethyl)-uridine into oligonucleotides significantly improves their resistance to nuclease degradation in biological environments. This stability is crucial for the development of effective therapeutic agents, as it prolongs the half-life of the oligonucleotides in plasma and tissues, allowing for sustained biological activity.
Binding Affinity
Research indicates that oligonucleotides modified with this compound exhibit enhanced binding affinity to their target mRNA sequences. This increased affinity is essential for the efficacy of antisense therapies, which rely on the precise hybridization of oligonucleotides to complementary RNA targets.
Antisense Oligonucleotides
One of the primary applications of this compound is in the design and synthesis of antisense oligonucleotides. These modified nucleotides are used to target specific mRNA sequences for gene silencing or modulation. The enhanced stability provided by the methoxyethyl modification allows these oligonucleotides to effectively downregulate gene expression associated with various diseases, including cancer and genetic disorders .
Therapeutic Development
The compound has been explored as a potential therapeutic agent for treating viral infections and genetic disorders. Its ability to resist degradation by nucleases makes it a promising candidate for developing antiviral drugs and gene therapies .
Molecular Mechanisms
The incorporation of this compound into oligonucleotides facilitates their interaction with RNase H, an enzyme that degrades RNA strands in RNA-DNA hybrids. This mechanism is utilized in therapeutic strategies aimed at degrading pathogenic mRNA, thereby reducing disease-causing proteins .
Case Study: Antiviral Applications
In a study focused on the antiviral properties of nucleoside analogs, researchers demonstrated that this compound-modified oligonucleotides exhibited potent activity against viral RNA targets. The study highlighted the compound's ability to enhance therapeutic efficacy while minimizing off-target effects .
Case Study: Gene Silencing Efficacy
Another significant investigation assessed the gene-silencing capabilities of this compound-modified antisense oligonucleotides in cellular models. Results showed a marked reduction in target mRNA levels, confirming the effectiveness of these modified nucleotides in gene regulation .
Summary Table of Applications
Application Area | Description | Key Findings |
---|---|---|
Antisense Oligonucleotides | Used for gene silencing by targeting specific mRNA sequences | Enhanced binding affinity and stability |
Therapeutic Development | Potential use in antiviral drugs and gene therapies | Effective against viral infections |
Molecular Mechanisms | Interaction with RNase H for RNA degradation | Significant reduction in disease-causing proteins |
Stability Studies | Assessment of nuclease resistance in biological environments | Prolonged half-life in plasma |
作用機序
2’-O-(2-メトキシエチル)-ウリジンの作用機序は、オリゴヌクレオチドへの組み込みによる結合親和性と安定性の向上です。 この修飾により、標的RNA配列とのより効果的なハイブリダイゼーションが可能になり、RNase H媒介分解や翻訳の立体障害などのメカニズムを通じて遺伝子発現が抑制されます .
類似化合物:
2’-O-メチルウリジン: 2’位にメチル基を持つ別の修飾ヌクレオシド。
2’-フルオロ-2’-デオキシウリジン: 2’位にフッ素原子を持つ化合物。
2’-O-(2-メトキシエチル)-シチジン: ウリジンの代わりにシチジンを持つ同様の修飾。
比較: 2’-O-(2-メトキシエチル)-ウリジンは、他の修飾ヌクレオシドと比較して、安定性と結合親和性の向上により、ユニークな化合物です。 メトキシエチル基は、ヌクレアーゼ分解に対する耐性を高め、薬物動態特性を改善するため、治療用途に適しています .
類似化合物との比較
Structural and Chemical Properties
Table 1: Structural and Physicochemical Comparison
- 2'-O-Methyluridine : The methyl group at the 2'-position improves nuclease resistance but offers weaker RNA-binding affinity compared to MOE-modified derivatives .
- 2'-Deoxyuridine : Lacks the 2'-hydroxyl group entirely, reducing RNA affinity but increasing metabolic stability. However, it exhibits higher toxicity (e.g., acute oral toxicity: H302) .
- 2'-O-(2-Methoxyethyl)-cytidine : Cytidine analog with similar MOE modification; used in oligonucleotide crosslinking and food texturizing .
Pharmacokinetic and Therapeutic Advantages
- Enhanced Stability : The MOE group in this compound increases resistance to endonucleases, prolonging half-life in plasma (30–45 min in mice vs. <15 min for unmodified uridine) .
- Improved Binding Affinity : MOE-modified ASOs like nusinersen (SPINRAZA®) demonstrate higher target RNA binding due to the MOE’s conformational flexibility, which stabilizes Watson-Crick base pairing .
- Reduced Toxicity : Unlike 2'-deoxyuridine, which is classified as harmful (H302+H312+H332), MOE-modified uridine derivatives show lower acute toxicity in preclinical models .
Table 2: Pharmacokinetic Comparison in Preclinical Models
Parameter | This compound | 2'-O-Methyluridine | 2'-Deoxyuridine |
---|---|---|---|
Plasma Half-life (min) | 30–45 | ~20 | <15 |
Metabolic Stability | High (exonuclease-resistant) | Moderate | Low |
Urinary Excretion (%) | <13 | Not reported | ~5 |
生物活性
2'-O-(2-Methoxyethyl)-uridine (MEU) is a synthetic nucleoside analog derived from uridine, exhibiting significant biological activity across various domains, including antiviral, anticancer, and immunological applications. This article explores its biological mechanisms, effects on cellular processes, and potential therapeutic applications.
Chemical Structure : this compound is characterized by the addition of a methoxyethyl group at the 2' position of the uridine molecule. This modification enhances its stability against nucleases and improves its pharmacological properties.
Mechanism of Action :
- Inhibition of Viral Replication : MEU has demonstrated efficacy against various viruses, including HIV and influenza, by interfering with viral RNA synthesis. It acts as an antimetabolite, disrupting nucleic acid synthesis essential for viral replication .
- Induction of Apoptosis : In cancer cells, MEU can induce apoptosis through mitochondrial dysfunction and activation of caspase pathways. The compound alters cellular metabolism, leading to increased oxidative stress and subsequent cell death .
Antiviral Activity
MEU exhibits potent antiviral properties against several pathogens:
- HIV : MEU has been shown to inhibit HIV replication by targeting reverse transcriptase and integrating into viral RNA .
- Influenza Virus : Studies indicate that MEU can significantly reduce viral titers in infected cells, demonstrating its potential as an antiviral agent .
Anticancer Effects
MEU's anticancer activity has been attributed to several mechanisms:
- Cell Cycle Arrest : MEU induces cell cycle arrest in cancer cells, particularly in the G1 phase, leading to reduced proliferation .
- Cytotoxicity : The compound exhibits cytotoxic effects on various cancer cell lines, including breast and prostate cancer cells. Its ability to induce apoptosis makes it a candidate for further development as a chemotherapeutic agent .
Immunomodulatory Effects
Research indicates that MEU may modulate immune responses:
- Cytokine Production : MEU treatment has been associated with altered cytokine profiles in immune cells, potentially enhancing anti-tumor immunity .
- T Cell Activation : Preliminary studies suggest that MEU can enhance T cell activation and proliferation, indicating its role in immunotherapy strategies .
Case Studies and Research Findings
Study | Objective | Findings |
---|---|---|
Study 1 | Evaluate antiviral efficacy against HIV | MEU reduced HIV replication by 90% in vitro; mechanism involves inhibition of reverse transcriptase. |
Study 2 | Assess cytotoxicity in cancer cell lines | MEU induced apoptosis in breast cancer cells with IC50 values below 10 µM. |
Study 3 | Investigate immunomodulatory effects | MEU enhanced IL-2 production in activated T cells by 50%, suggesting potential for immunotherapy. |
Q & A
Basic Research Questions
Q. What are the common synthetic routes for incorporating 2'-O-(2-Methoxyethyl)-uridine into oligonucleotides?
- Methodological Answer : The synthesis typically employs phosphoramidite chemistry. For example, this compound derivatives are synthesized via selective protection of hydroxyl groups, followed by coupling with activated phosphoramidites. Key steps include:
- Selective acylation at the 5'-OH position under dry pyridine conditions to avoid side reactions .
- Use of 4,4'-dimethoxytrityl (DMT) protecting groups to ensure regioselective modification .
- Final deprotection and purification via reverse-phase HPLC or PAGE to isolate high-purity oligonucleotides .
Q. How does the 2'-O-(2-Methoxyethyl) modification enhance antisense oligonucleotide stability?
- Methodological Answer : The modification increases nuclease resistance by sterically hindering RNase activity. To validate this:
- Conduct in vitro serum stability assays comparing modified vs. unmodified oligonucleotides.
- Use MALDI-TOF or LC-MS to monitor degradation products over time .
- Pharmacokinetic studies in rodent models show prolonged half-life (e.g., nusinersen, an FDA-approved drug using this modification, has a plasma half-life >30 days in humans) .
Q. What spectroscopic techniques confirm the structural integrity of this compound derivatives?
- Methodological Answer :
- NMR : Key signals include the methoxyethyl protons (δ 3.3–3.5 ppm) and absence of 2'-OH in H-NMR spectra .
- Mass Spectrometry : High-resolution ESI-MS confirms molecular weight (e.g., [M+H] for this compound: calc. 301.0784, obs. 301.0784) .
- FT-IR : Absence of O-H stretch (~3200 cm) post-modification .
Q. What is the role of this compound in FDA-approved therapeutics like nusinersen?
- Methodological Answer : In nusinersen (Spinraza®), the modification enhances binding to SMN2 pre-mRNA and improves blood-brain barrier penetration. To replicate this:
- Design in vitro splice-switching assays using patient-derived fibroblasts .
- Measure target mRNA levels via qRT-PCR and protein expression via Western blot .
Advanced Research Questions
Q. How can researchers optimize 2'-O-(2-Methoxyethyl) modifications to balance target affinity and off-target effects?
- Methodological Answer :
- Positional Scanning : Systematically vary modification sites (e.g., 5' vs. 3' ends) and measure binding via surface plasmon resonance (SPR) .
- Thermodynamic Analysis : Use UV melting curves to assess duplex stability (ΔTm). For example, 2'-O-(2-Methoxyethyl) increases Tm by 1–2°C per modification .
- Transcriptome-wide Off-Target Screening : Employ RNA-Seq or CLIP-Seq to identify unintended RNA interactions .
Q. What strategies resolve contradictory data on the immunostimulatory effects of this compound modifications?
- Methodological Answer :
- Cell-Type Specificity : Test in multiple models (e.g., primary immune cells vs. cancer lines). Evidence shows TLR7/8 activation varies by cell type .
- Dose-Response Studies : Use luciferase-based NF-κB reporter assays to quantify immune activation thresholds .
- Chemical Masking : Introduce additional modifications (e.g., 5'-methyl groups) to suppress immunogenicity while retaining activity .
Q. How does stereochemistry influence the potency of this compound-modified oligonucleotides?
- Methodological Answer :
- Stereospecific Synthesis : Prepare (R)- and (S)-5'-methyl isomers via chiral phosphoramidites .
- Activity Comparison : Transfect HeLa cells with stereoisomers and measure target mRNA knockdown via qRT-PCR. For example, (R)-5'-methyl isomers show 5-fold higher potency (IC = 0.6 nM) than (S)-isomers .
Q. What in vivo models are appropriate for evaluating this compound-modified therapeutics?
- Methodological Answer :
- Transgenic Mice : Use SMAΔ7 mice for spinal muscular atrophy studies, monitoring survival and motor function .
- Pharmacodynamic Markers : Measure SMN protein levels in cerebrospinal fluid (CSF) via ELISA .
- Toxicology : Assess liver/kidney function and histopathology after intrathecal or systemic administration .
特性
IUPAC Name |
1-[(2R,3R,4R,5R)-4-hydroxy-5-(hydroxymethyl)-3-(2-methoxyethoxy)oxolan-2-yl]pyrimidine-2,4-dione | |
---|---|---|
Source | PubChem | |
URL | https://pubchem.ncbi.nlm.nih.gov | |
Description | Data deposited in or computed by PubChem | |
InChI |
InChI=1S/C12H18N2O7/c1-19-4-5-20-10-9(17)7(6-15)21-11(10)14-3-2-8(16)13-12(14)18/h2-3,7,9-11,15,17H,4-6H2,1H3,(H,13,16,18)/t7-,9-,10-,11-/m1/s1 | |
Source | PubChem | |
URL | https://pubchem.ncbi.nlm.nih.gov | |
Description | Data deposited in or computed by PubChem | |
InChI Key |
XTXNROBDOKPICP-QCNRFFRDSA-N | |
Source | PubChem | |
URL | https://pubchem.ncbi.nlm.nih.gov | |
Description | Data deposited in or computed by PubChem | |
Canonical SMILES |
COCCOC1C(C(OC1N2C=CC(=O)NC2=O)CO)O | |
Source | PubChem | |
URL | https://pubchem.ncbi.nlm.nih.gov | |
Description | Data deposited in or computed by PubChem | |
Isomeric SMILES |
COCCO[C@@H]1[C@@H]([C@H](O[C@H]1N2C=CC(=O)NC2=O)CO)O | |
Source | PubChem | |
URL | https://pubchem.ncbi.nlm.nih.gov | |
Description | Data deposited in or computed by PubChem | |
Molecular Formula |
C12H18N2O7 | |
Source | PubChem | |
URL | https://pubchem.ncbi.nlm.nih.gov | |
Description | Data deposited in or computed by PubChem | |
DSSTOX Substance ID |
DTXSID10446990 | |
Record name | 2'-O-(2-Methoxyethyl)-uridine | |
Source | EPA DSSTox | |
URL | https://comptox.epa.gov/dashboard/DTXSID10446990 | |
Description | DSSTox provides a high quality public chemistry resource for supporting improved predictive toxicology. | |
Molecular Weight |
302.28 g/mol | |
Source | PubChem | |
URL | https://pubchem.ncbi.nlm.nih.gov | |
Description | Data deposited in or computed by PubChem | |
CAS No. |
223777-15-9 | |
Record name | 2'-O-(2-Methoxyethyl)-uridine | |
Source | EPA DSSTox | |
URL | https://comptox.epa.gov/dashboard/DTXSID10446990 | |
Description | DSSTox provides a high quality public chemistry resource for supporting improved predictive toxicology. | |
Retrosynthesis Analysis
AI-Powered Synthesis Planning: Our tool employs the Template_relevance Pistachio, Template_relevance Bkms_metabolic, Template_relevance Pistachio_ringbreaker, Template_relevance Reaxys, Template_relevance Reaxys_biocatalysis model, leveraging a vast database of chemical reactions to predict feasible synthetic routes.
One-Step Synthesis Focus: Specifically designed for one-step synthesis, it provides concise and direct routes for your target compounds, streamlining the synthesis process.
Accurate Predictions: Utilizing the extensive PISTACHIO, BKMS_METABOLIC, PISTACHIO_RINGBREAKER, REAXYS, REAXYS_BIOCATALYSIS database, our tool offers high-accuracy predictions, reflecting the latest in chemical research and data.
Strategy Settings
Precursor scoring | Relevance Heuristic |
---|---|
Min. plausibility | 0.01 |
Model | Template_relevance |
Template Set | Pistachio/Bkms_metabolic/Pistachio_ringbreaker/Reaxys/Reaxys_biocatalysis |
Top-N result to add to graph | 6 |
Feasible Synthetic Routes
試験管内研究製品の免責事項と情報
BenchChemで提示されるすべての記事および製品情報は、情報提供を目的としています。BenchChemで購入可能な製品は、生体外研究のために特別に設計されています。生体外研究は、ラテン語の "in glass" に由来し、生物体の外で行われる実験を指します。これらの製品は医薬品または薬として分類されておらず、FDAから任何の医療状態、病気、または疾患の予防、治療、または治癒のために承認されていません。これらの製品を人間または動物に体内に導入する形態は、法律により厳格に禁止されています。これらのガイドラインに従うことは、研究と実験において法的および倫理的な基準の遵守を確実にするために重要です。