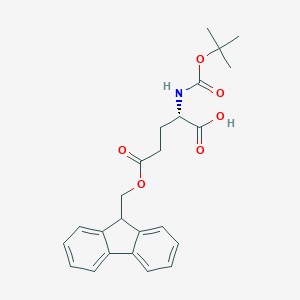
Boc-Glu(Ofm)-OH
概要
説明
科学的研究の応用
Boc-glu(ofm)-oh is widely used in scientific research, particularly in the fields of chemistry, biology, medicine, and industry .
Chemistry: It is used in the synthesis of complex peptides and proteins, including cyclic peptides and ester insulin.
Biology: The compound is used in the study of protein-protein interactions and enzyme-substrate interactions.
Medicine: this compound is used in the development of peptide-based drugs and therapeutic agents.
Industry: It is used in the production of peptide-based materials and bioconjugates.
作用機序
Target of Action
Boc-Glu(Ofm)-OH is a peptide that has been used for the synthesis of ester insulin and cyclic peptide mixtures . The primary targets of this compound are therefore the insulin receptors and the receptors of the cyclic peptides it helps synthesize.
Mode of Action
This change then triggers a cascade of intracellular events, leading to the desired biological response .
Biochemical Pathways
The biochemical pathways affected by this compound are likely those involved in glucose metabolism (in the case of ester insulin) and the specific pathways targeted by the cyclic peptides it helps synthesize. The downstream effects of these pathways can vary widely depending on the specific cyclic peptide being synthesized .
Pharmacokinetics
Its bioavailability would also be influenced by factors such as its route of administration and the presence of any modifications that increase its stability .
Result of Action
The molecular and cellular effects of this compound’s action would depend on the specific ester insulin or cyclic peptide it helps synthesize. For instance, if it is used to synthesize ester insulin, the result of its action would be the regulation of glucose metabolism in the body .
Action Environment
The action, efficacy, and stability of this compound can be influenced by various environmental factors. These may include the pH and temperature of its surroundings, the presence of other interacting molecules, and the specific cellular environment in which it is present .
生化学分析
Biochemical Properties
It is known that Boc-glu(ofm)-oh has been used for the synthesis of ester insulin and cyclic peptide mixtures . This suggests that it may interact with enzymes, proteins, and other biomolecules involved in these processes.
Cellular Effects
Given its use in the synthesis of ester insulin and cyclic peptide mixtures , it may influence cell function, including impacts on cell signaling pathways, gene expression, and cellular metabolism.
Molecular Mechanism
Given its use in the synthesis of ester insulin and cyclic peptide mixtures , it may exert its effects at the molecular level, including binding interactions with biomolecules, enzyme inhibition or activation, and changes in gene expression.
準備方法
Synthetic Routes and Reaction Conditions
The synthesis of Boc-glu(ofm)-oh typically involves the protection of the amino group with a tert-butoxycarbonyl (Boc) group and the carboxyl group with a fluorenylmethoxycarbonyl (Fmoc) group. The synthesis can be carried out using solid-phase peptide synthesis (SPPS) techniques, where the peptide is assembled step-by-step on a solid support .
Protection of the Amino Group: The amino group of glutamic acid is protected using the Boc group.
Protection of the Carboxyl Group: The carboxyl group is protected using the Fmoc group.
Coupling Reactions: The protected amino acid is then coupled with other amino acids or peptides using coupling reagents like dicyclohexylcarbodiimide (DCC) or N,N’-diisopropylcarbodiimide (DIC).
Deprotection: The Boc and Fmoc groups are removed using trifluoroacetic acid (TFA) and piperidine, respectively.
Industrial Production Methods
Industrial production of this compound follows similar synthetic routes but on a larger scale. The use of automated peptide synthesizers and high-throughput techniques allows for efficient and large-scale production of this compound .
化学反応の分析
Types of Reactions
Boc-glu(ofm)-oh undergoes various chemical reactions, including:
Oxidation: The compound can be oxidized to form disulfide bonds in peptides.
Reduction: Reduction reactions can be used to break disulfide bonds.
Substitution: The Boc and Fmoc groups can be substituted with other protecting groups or functional groups.
Common Reagents and Conditions
Oxidation: Hydrogen peroxide (H₂O₂) or iodine (I₂) can be used as oxidizing agents.
Reduction: Dithiothreitol (DTT) or tris(2-carboxyethyl)phosphine (TCEP) are common reducing agents.
Substitution: TFA and piperidine are used for deprotection reactions.
Major Products Formed
The major products formed from these reactions include deprotected peptides, cyclic peptides, and ester insulin .
類似化合物との比較
Boc-glu(ofm)-oh is unique due to its dual protection of both the amino and carboxyl groups, which allows for greater control and precision in peptide synthesis. Similar compounds include:
Boc-glu(otbu)-oh: This compound uses a tert-butyl (tBu) group instead of the Fmoc group for carboxyl protection.
Fmoc-glu(ofm)-oh: This compound uses an Fmoc group for amino protection instead of the Boc group.
Boc-ala-oh: This compound is a simpler derivative with only the Boc group for amino protection
These compounds differ in their protecting groups and their specific applications in peptide synthesis.
生物活性
Boc-Glu(OFm)-OH, also known as N-α-tert-butoxycarbonyl-D-glutamic acid α-9-fluorenylmethyl ester, is a synthetic compound primarily utilized in peptide synthesis. Its unique structure allows it to play significant roles in various biological applications, particularly in the development of peptide-based therapeutics and insulin analogs. This article explores the biological activity of this compound, including its mechanisms of action, biochemical pathways, and applications in research.
Chemical Structure and Synthesis
This compound features a tert-butoxycarbonyl (Boc) protecting group on the amino group of D-glutamic acid and a fluorenylmethoxycarbonyl (Fmoc) protecting group on the carboxyl group. The synthesis typically involves:
- Protection of the Amino Group : The amino group is protected using Boc anhydride.
- Protection of the Carboxyl Group : The carboxyl group is protected using Fmoc chloride.
- Coupling Reactions : The protected amino acid can be coupled with other amino acids or peptides using coupling agents like dicyclohexylcarbodiimide (DCC) or N,N’-diisopropylcarbodiimide (DIC) .
This compound does not exhibit direct biological activity on its own; rather, it serves as a building block for more complex peptides that can interact with various biological targets. The mechanism of action is largely dependent on the specific peptides synthesized from it, which can modulate biochemical pathways involved in:
- Glucose Metabolism : When used in the synthesis of ester insulin, this compound influences insulin signaling pathways, thereby regulating glucose homeostasis .
- Protein-Protein Interactions : The cyclic peptides formed from this compound can affect interactions between proteins, impacting cellular signaling .
The compound has been shown to reversibly inhibit purine nucleoside phosphorylase, an enzyme involved in purine metabolism. This inhibition can affect cellular proliferation and apoptosis pathways . Additionally, its stability and bioavailability are influenced by environmental factors such as pH and temperature during synthesis and application .
Applications in Research
This compound has several significant applications across various fields:
- Peptide Synthesis : It is extensively used in solid-phase peptide synthesis (SPPS), allowing for the creation of complex peptide structures necessary for therapeutic agents .
- Insulin Analog Development : The compound is integral in synthesizing ester insulin, which has implications for diabetes treatment by providing alternative forms of insulin with modified pharmacokinetics .
- Drug Development : Its role in synthesizing cyclic peptides makes it valuable for developing new peptide-based drugs targeting specific receptors or enzymes .
Case Studies
- Ester Insulin Synthesis : Research demonstrated that this compound was crucial in synthesizing ester-linked dipeptides that serve as precursors for insulin analogs. These analogs showed improved stability and bioactivity compared to traditional insulin formulations .
- Cyclic Peptide Research : In studies involving cyclic peptides derived from this compound, researchers found enhanced binding affinities to specific receptors compared to their linear counterparts, indicating potential therapeutic benefits .
Comparison with Similar Compounds
This compound can be compared with other similar compounds based on their protective groups and applications:
Compound | Amino Protection | Carboxyl Protection | Application |
---|---|---|---|
This compound | Boc | Fmoc | Peptide synthesis |
Fmoc-Glu(OFm)-OH | Fmoc | Fmoc | Peptide synthesis |
Boc-Glu(OtBu)-OH | Boc | tBu | Alternative peptide synthesis |
特性
IUPAC Name |
(2S)-5-(9H-fluoren-9-ylmethoxy)-2-[(2-methylpropan-2-yl)oxycarbonylamino]-5-oxopentanoic acid | |
---|---|---|
Source | PubChem | |
URL | https://pubchem.ncbi.nlm.nih.gov | |
Description | Data deposited in or computed by PubChem | |
InChI |
InChI=1S/C24H27NO6/c1-24(2,3)31-23(29)25-20(22(27)28)12-13-21(26)30-14-19-17-10-6-4-8-15(17)16-9-5-7-11-18(16)19/h4-11,19-20H,12-14H2,1-3H3,(H,25,29)(H,27,28)/t20-/m0/s1 | |
Source | PubChem | |
URL | https://pubchem.ncbi.nlm.nih.gov | |
Description | Data deposited in or computed by PubChem | |
InChI Key |
RCQRXYWDDVULAP-FQEVSTJZSA-N | |
Source | PubChem | |
URL | https://pubchem.ncbi.nlm.nih.gov | |
Description | Data deposited in or computed by PubChem | |
Canonical SMILES |
CC(C)(C)OC(=O)NC(CCC(=O)OCC1C2=CC=CC=C2C3=CC=CC=C13)C(=O)O | |
Source | PubChem | |
URL | https://pubchem.ncbi.nlm.nih.gov | |
Description | Data deposited in or computed by PubChem | |
Isomeric SMILES |
CC(C)(C)OC(=O)N[C@@H](CCC(=O)OCC1C2=CC=CC=C2C3=CC=CC=C13)C(=O)O | |
Source | PubChem | |
URL | https://pubchem.ncbi.nlm.nih.gov | |
Description | Data deposited in or computed by PubChem | |
Molecular Formula |
C24H27NO6 | |
Source | PubChem | |
URL | https://pubchem.ncbi.nlm.nih.gov | |
Description | Data deposited in or computed by PubChem | |
DSSTOX Substance ID |
DTXSID80154010 | |
Record name | N-alpha-tert-Butyloxycarbonylglutamic acid gamma-fluorenylmethyl ester | |
Source | EPA DSSTox | |
URL | https://comptox.epa.gov/dashboard/DTXSID80154010 | |
Description | DSSTox provides a high quality public chemistry resource for supporting improved predictive toxicology. | |
Molecular Weight |
425.5 g/mol | |
Source | PubChem | |
URL | https://pubchem.ncbi.nlm.nih.gov | |
Description | Data deposited in or computed by PubChem | |
CAS No. |
123417-18-5 | |
Record name | N-alpha-tert-Butyloxycarbonylglutamic acid gamma-fluorenylmethyl ester | |
Source | ChemIDplus | |
URL | https://pubchem.ncbi.nlm.nih.gov/substance/?source=chemidplus&sourceid=0123417185 | |
Description | ChemIDplus is a free, web search system that provides access to the structure and nomenclature authority files used for the identification of chemical substances cited in National Library of Medicine (NLM) databases, including the TOXNET system. | |
Record name | N-alpha-tert-Butyloxycarbonylglutamic acid gamma-fluorenylmethyl ester | |
Source | EPA DSSTox | |
URL | https://comptox.epa.gov/dashboard/DTXSID80154010 | |
Description | DSSTox provides a high quality public chemistry resource for supporting improved predictive toxicology. | |
Retrosynthesis Analysis
AI-Powered Synthesis Planning: Our tool employs the Template_relevance Pistachio, Template_relevance Bkms_metabolic, Template_relevance Pistachio_ringbreaker, Template_relevance Reaxys, Template_relevance Reaxys_biocatalysis model, leveraging a vast database of chemical reactions to predict feasible synthetic routes.
One-Step Synthesis Focus: Specifically designed for one-step synthesis, it provides concise and direct routes for your target compounds, streamlining the synthesis process.
Accurate Predictions: Utilizing the extensive PISTACHIO, BKMS_METABOLIC, PISTACHIO_RINGBREAKER, REAXYS, REAXYS_BIOCATALYSIS database, our tool offers high-accuracy predictions, reflecting the latest in chemical research and data.
Strategy Settings
Precursor scoring | Relevance Heuristic |
---|---|
Min. plausibility | 0.01 |
Model | Template_relevance |
Template Set | Pistachio/Bkms_metabolic/Pistachio_ringbreaker/Reaxys/Reaxys_biocatalysis |
Top-N result to add to graph | 6 |
Feasible Synthetic Routes
試験管内研究製品の免責事項と情報
BenchChemで提示されるすべての記事および製品情報は、情報提供を目的としています。BenchChemで購入可能な製品は、生体外研究のために特別に設計されています。生体外研究は、ラテン語の "in glass" に由来し、生物体の外で行われる実験を指します。これらの製品は医薬品または薬として分類されておらず、FDAから任何の医療状態、病気、または疾患の予防、治療、または治癒のために承認されていません。これらの製品を人間または動物に体内に導入する形態は、法律により厳格に禁止されています。これらのガイドラインに従うことは、研究と実験において法的および倫理的な基準の遵守を確実にするために重要です。