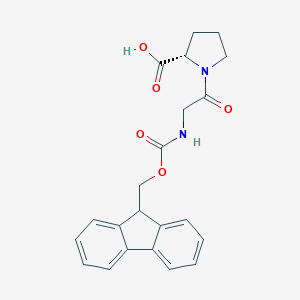
Fmoc-Gly-Pro-OH
概要
説明
Fmoc-Gly-Pro-OH is a protected dipeptide derivative widely used in solid-phase peptide synthesis (SPPS). The compound consists of glycine (Gly) and proline (Pro) residues, with the N-terminal glycine protected by a 9-fluorenylmethyloxycarbonyl (Fmoc) group. Key properties include:
- Molecular formula: C₂₂H₂₂N₂O₅
- Molecular weight: 394.43 g/mol
- Solubility: Soluble in DMSO; enhanced via heating (37°C) and sonication
- Purity: >98.00% (HPLC)
- Storage: Stable at -20°C for 1 month or -80°C for 6 months in solution
This compound is critical for constructing peptide backbones, particularly in collagen mimetics and bioactive peptides, due to Pro’s rigid pyrrolidine ring, which influences secondary structure formation .
準備方法
Solid-Phase Peptide Synthesis (SPPS) of Fmoc-Gly-Pro-OH
Standard Fmoc-SPPS Protocol
The majority of this compound synthesis employs SPPS due to its high efficiency and scalability. A typical protocol involves the sequential coupling of Fmoc-protected amino acids to a resin-bound peptide chain. The process begins with the attachment of Fmoc-Pro-OH to a rink amide resin, followed by deprotection using 20–40% piperidine in DMF to remove the Fmoc group . Glycine is then coupled using activating agents such as HBTU/HOBt or HATU, with DIPEA as a base . Reaction conditions are tightly controlled, with temperatures ranging from 25°C to 40°C and coupling times of 40 minutes to 2 hours .
Critical Parameters:
-
Resin Swelling: DMF is used to swell the resin for 15 minutes prior to synthesis .
-
Coupling Agents: Double couplings with 5 equivalents of Fmoc-Gly-OH ensure >99% yield .
-
Deprotection Efficiency: Piperidine concentration (20–40%) and reaction time (3–12 minutes) balance completeness and side reactions .
Table 1: SPPS Conditions for this compound from Select Studies
Microwave-Assisted SPPS
Microwave irradiation significantly accelerates this compound synthesis. Studies demonstrate that 50–100 W irradiation reduces coupling times from 40 minutes to 10–20 seconds while maintaining >98% purity . The protocol involves preactivating Fmoc-Pro-OH with PyBOP/DIPEA before microwave-assisted coupling to the glycine-resin intermediate. Careful temperature control (10°C cooling between cycles) prevents racemization .
Solution-Phase Synthesis and Fragment Condensation
Fragment Condensation Approach
Industrial-scale production often employs fragment condensation to avoid resin-related limitations. Fmoc-Gly-OH and Fmoc-Pro-OH are preactivated separately using DIC/HOBt, then combined in anhydrous DCM at 0°C . This method achieves 85–92% yields but requires stringent purification due to dipeptide aggregation tendencies .
Continuous Flow Synthesis
Recent advancements utilize flow chemistry for this compound synthesis. A stainless steel reactor maintained at 60°C enables rapid (3-minute) coupling cycles with 1 mmol Fmoc-amino acids and HBTU/DIEA . This method achieves 95% conversion efficiency and reduces solvent usage by 40% compared to batch processes .
Critical Challenges and Optimization Strategies
Racemization Control
Glycine’s lack of a chiral center simplifies synthesis, but proline’s secondary amine increases racemization risk. Strategies include:
-
Low-Temperature Coupling: Maintaining reactions at 10°C reduces epimerization .
-
Optimized Base Concentration: DIEA concentrations ≤6 equivalents minimize base-induced racemization .
-
Trityl Protection: Using Fmoc-Pro(Trt)-OH instead of Boc protection reduces side reactions during SPPS .
Purification and Analysis
Crude this compound is purified via reverse-phase HPLC using C18 columns and acetonitrile/water gradients . Mass spectrometry (ESI-TOF) confirms molecular integrity, with typical [M+H]+ peaks at m/z 425.2 . Purity ≥99% is achievable through iterative washes with cold diethyl ether and lyophilization .
Industrial-Scale Production Insights
Cost-Effective Dipeptide Utilization
The patent WO2010117725 highlights the use of preassembled Fmoc-Gly-Gly-OH and Fmoc-Pro-Pro-OH dipeptides to reduce synthesis costs by 30% . This approach minimizes coupling steps and improves batch consistency in large-scale runs.
Green Chemistry Innovations
Replacing DMF with cyclopentyl methyl ether (CPME) in SPPS reduces environmental impact while maintaining 90% coupling efficiency . Additionally, piperidine recycling systems cut solvent waste by 50% in industrial facilities .
化学反応の分析
Types of Reactions: Fmoc-Gly-Pro-OH undergoes various chemical reactions, including:
Deprotection: The Fmoc group can be removed under basic conditions, typically using piperidine in DMF.
Coupling Reactions: The compound can participate in peptide bond formation with other amino acids or peptides.
Hydrolysis: The ester bond in the Fmoc group can be hydrolyzed under acidic or basic conditions.
Common Reagents and Conditions:
Deprotection: Piperidine in DMF (20% solution) is commonly used.
Coupling: DIC and HOBt in DMF are typical reagents.
Hydrolysis: Acidic or basic conditions, depending on the desired outcome.
Major Products:
Deprotection: Removal of the Fmoc group yields the free amine form of the peptide.
Coupling: Formation of extended peptide chains.
Hydrolysis: Cleavage of the ester bond results in the formation of free carboxylic acids.
科学的研究の応用
Peptide Synthesis
Overview : Fmoc-Gly-Pro-OH is predominantly used in solid-phase peptide synthesis (SPPS), which allows for the efficient assembly of peptides. The Fmoc (9-fluorenylmethyloxycarbonyl) group provides stability during the synthesis process, facilitating the formation of complex peptide structures.
Key Benefits :
- Enhances yield and purity of synthesized peptides.
- Reduces aggregation issues often encountered with peptides.
Drug Development
Role in Pharmaceuticals : This compound is instrumental in developing peptide-based drugs. Its stable structure contributes to improved bioavailability and pharmacological efficacy.
Case Studies :
- Peptide Therapeutics : Research has shown that incorporating this compound into drug candidates can enhance their therapeutic profiles, particularly in targeting specific biological pathways .
Bioconjugation
Application : this compound is utilized in bioconjugation processes, which involve attaching biomolecules to surfaces or other molecules. This enhances the functionality of diagnostic tools and therapeutic agents.
Impact :
- Improves the stability and effectiveness of conjugated biomolecules.
- Facilitates the development of advanced drug delivery systems.
Protein Engineering
Significance : Researchers leverage this compound to design and modify proteins, which is crucial for studying protein interactions and functions.
Insights :
- Studies have demonstrated that peptides containing this compound can stabilize protein structures, thereby aiding in understanding their biological functions .
Research in Neuroscience
Focus Area : The compound is applied in studies related to neuropeptides, contributing to the understanding of signaling pathways involved in neurological disorders.
Findings :
- Investigations have linked modifications using this compound to enhanced neuropeptide activity, potentially leading to novel treatments for conditions such as depression and anxiety .
Case Study 1: Peptide Therapeutics
A study highlighted the use of this compound in synthesizing a peptide that demonstrated significant anti-inflammatory properties when tested in vivo. The incorporation of this compound improved the peptide's stability and efficacy compared to traditional methods .
Case Study 2: Protein Interaction Studies
Research involving this compound revealed its effectiveness in elucidating protein-protein interactions. The modified peptides provided clearer results due to reduced aggregation, allowing for more accurate binding studies .
作用機序
The mechanism of action of Fmoc-Gly-Pro-OH primarily involves its role as a building block in peptide synthesis. The Fmoc group protects the amino terminus, allowing for selective reactions at other functional groups. Upon deprotection, the free amine can participate in further coupling reactions, facilitating the formation of peptide bonds.
類似化合物との比較
Positional Isomer: Fmoc-Pro-Gly-OH
- Structure : Reversed sequence (Pro-Gly vs. Gly-Pro) .
- Molecular formula : C₂₂H₂₂N₂O₅ (identical to Fmoc-Gly-Pro-OH) .
- CAS No.: 250695-65-9 .
- Purity : 97% .
- Limited data on biological activity compared to this compound, though sequence inversion may alter protease resistance .
Extended Chain: Fmoc-Gly-Pro-Pro-OH
- Structure : Gly-Pro-Pro tripeptide with Fmoc protection .
- Molecular formula : C₂₇H₃₂N₃O₆ .
- Molecular weight : 492.21 g/mol (calculated) .
- Synthesis :
- Applications : Used in collagen mimetic studies due to Pro’s propensity for polyproline helices .
Property | This compound | Fmoc-Gly-Pro-Pro-OH |
---|---|---|
Molecular Weight | 394.43 | 492.21 |
Solubility | DMSO | DMSO/THF mixtures |
Structural Role | Dipeptide | Tripeptide helix |
Hydroxyproline Derivative: Fmoc-Gly-Pro-Hyp-OH
- Structure : Gly-Pro-Hyp tripeptide with Hyp (hydroxyproline) replacing Pro at the third position .
- Molecular formula : C₂₇H₂₉N₃O₇ .
- Molecular weight : 507.54 g/mol .
- Key differences :
Protecting Group Variant: Boc-Pro-Gly-OH
- Structure : Pro-Gly dipeptide with tert-butoxycarbonyl (Boc) protection instead of Fmoc .
- Key differences :
Ferrocene Conjugate: Fc-Gly-Pro-Arg(NO₂)-OMe
- Structure : Ferrocene (Fc)-tagged Gly-Pro-Arg tripeptide with a nitro group .
- Synthesis :
- Applications: Electrochemical studies with Cu(II), forming a 2:1 Fc-GPR:Cu(II) complex . Potential therapeutic relevance in Alzheimer’s disease due to interactions with β-amyloid peptides .
Property | This compound | Fc-Gly-Pro-Arg(NO₂)-OMe |
---|---|---|
Molecular Weight | 394.43 | ~800 (estimated) |
Function | Peptide synthesis | Metal ion coordination |
Therapeutic Potential | Limited | Alzheimer’s research |
生物活性
Fmoc-Gly-Pro-OH, or 9-fluorenylmethoxycarbonyl-glycine-proline, is a synthetic compound widely utilized in peptide synthesis. It combines a glycine residue with a proline residue, with the Fmoc group serving as a protective group for the amino functionality during the synthesis process. This article explores the biological activity of this compound, focusing on its structural components, potential roles in biological systems, and relevant research findings.
Chemical Structure and Properties
- Molecular Formula : C22H22N2O5
- Molecular Weight : Approximately 394.42 g/mol
- Structure : The compound features a glycine and proline linkage, with the Fmoc group providing stability and ease of handling during synthesis.
Biological Significance
While specific biological activities of this compound are not extensively documented, its structural components suggest several potential roles in biological systems:
- Peptide Synthesis : this compound is primarily used as a building block in solid-phase peptide synthesis (SPPS), allowing for the controlled assembly of peptides with specific sequences and functionalities.
- Collagen Synthesis : Glycine and proline are known to be critical in collagen formation, which is essential for maintaining structural integrity in various tissues.
- Conformational Stability : The presence of proline can influence the conformation and stability of peptides, potentially affecting their biological functions such as cell signaling and structural integrity of proteins.
1. Structural Activity Relationship Studies
Research indicates that the incorporation of proline significantly influences peptide conformation and stability. Techniques like nuclear magnetic resonance (NMR) spectroscopy and circular dichroism (CD) are commonly employed to analyze conformational changes upon binding or interaction with other biomolecules.
2. Interaction Studies
Studies have shown that peptides containing proline exhibit unique folding patterns and stability characteristics compared to those lacking proline. For example, interactions between this compound and various biomolecules have been investigated to understand its role in peptide behavior.
3. Diketopiperazine Formation
A mechanistic study highlighted the formation of diketopiperazine (DKP) during solid-phase synthesis involving Fmoc-Pro-OH derivatives. The research indicated that prolonged deprotection times could lead to increased DKP formation, which may affect the purity and biological activity of synthesized peptides .
Case Study 1: Peptide Synthesis Applications
A study utilized this compound in the development of polymer-based DNA delivery systems. The research emphasized how oligopeptide orientation influenced delivery efficacy, showcasing the importance of peptide design in therapeutic applications .
Case Study 2: Role in Collagen Studies
Investigations into compounds similar to this compound, such as Fmoc-Gly-Pro-Hyp-OH (which includes hydroxyproline), have been conducted to understand their relevance in collagen studies. These studies underline the significance of proline derivatives in enhancing collagen stability and functionality .
Comparative Analysis Table
Compound Name | Structure | Unique Features |
---|---|---|
Fmoc-Gly-OH | C21H19N2O4 | Lacks proline; simpler structure for basic peptides |
Fmoc-Pro-OH | C21H19N2O4 | Contains only proline; useful for specific applications |
Fmoc-Gly-Pro-Hyp-OH | C22H22N2O5 | Contains hydroxyproline; relevant in collagen studies |
Fmoc-Ala-Pro-OH | C22H24N2O4 | Contains alanine; alters hydrophobicity |
Q & A
Basic Questions
Q. How should researchers determine the optimal solvent for preparing Fmoc-Gly-Pro-OH stock solutions?
this compound is typically soluble in DMSO, but solubility varies with solvent polarity and temperature. To prepare stock solutions:
- Use DMSO as the primary solvent due to its high solubility for Fmoc-protected peptides .
- If solubility is insufficient, heat the solution to 37°C and sonicate in an ultrasonic bath to disperse aggregates .
- Avoid aqueous buffers unless coupled with co-solvents (e.g., PEG300, Tween 80) for in vivo applications .
Q. What storage conditions are critical for maintaining this compound stability?
- Short-term storage : Store lyophilized powder at -20°C in a desiccator to prevent hydrolysis .
- Long-term storage : Dissolved stock solutions should be aliquoted and stored at -80°C for up to 6 months; avoid repeated freeze-thaw cycles to prevent degradation .
- Monitor purity via HPLC (>98% by QC data) before critical experiments .
Q. How can researchers validate the structural integrity of this compound before use?
- Analytical HPLC : Use a C18 column with a gradient of acetonitrile/water (0.1% TFA) to confirm retention time and peak homogeneity .
- Mass spectrometry (MS) : Verify molecular weight (expected: 394.42 g/mol) using ESI-MS or MALDI-TOF .
- NMR : Compare ¹H/¹³C spectra with published data to confirm absence of stereochemical impurities .
Advanced Research Questions
Q. What strategies mitigate steric hindrance during this compound incorporation into peptide chains?
Proline’s cyclic structure introduces conformational constraints. To improve coupling efficiency:
- Use double coupling protocols with activators like HBTU/HOBt in DMF .
- Extend reaction times (30–60 minutes) and monitor by Kaiser test for unreacted amines .
- For challenging sequences, employ microwave-assisted synthesis to enhance reaction kinetics .
Q. How can in vivo formulations of this compound be optimized for bioavailability?
- Solubility enhancement : Prepare a co-solvent mixture of 5% DMSO, 30% PEG300, 5% Tween 80, and 60% saline. Add solvents sequentially to ensure clarity .
- Dosing calculations : For a 20 g mouse, dissolve 2 mg of compound in 100 µL DMSO (20 mg/mL stock), then dilute to 2 mg/mL with the co-solvent mixture for a 10 mg/kg dose .
- Validate biocompatibility via preliminary toxicity assays (e.g., hemolysis tests) .
Q. What analytical methods resolve contradictions in reported purity data for this compound?
- Orthogonal validation : Cross-check HPLC purity (>98%) with LC-MS to detect trace impurities (e.g., deprotected glycine or proline residues) .
- Quantitative NMR (qNMR) : Use an internal standard (e.g., maleic acid) to quantify absolute purity and correct for solvent-related discrepancies .
- Batch-to-batch consistency : Request COA (Certificate of Analysis) from suppliers to compare retention times and impurity profiles .
Q. How does the proline residue in this compound influence peptide secondary structure?
Proline’s trans-amide bond preference disrupts α-helix formation but stabilizes β-turns. To study structural impacts:
- Perform circular dichroism (CD) spectroscopy in aqueous and membrane-mimetic environments .
- Use molecular dynamics simulations to model conformational flexibility in peptide backbones .
- Compare with analogs (e.g., Fmoc-Ala-Pro-OH) to isolate proline-specific effects .
Q. Methodological Notes
- Synthesis protocols : Follow Fmoc-SPPS (solid-phase peptide synthesis) with Rink amide resin for C-terminal amidation .
- Degradation prevention : Store lyophilized peptide under argon to minimize oxidation of the Fmoc group .
- Safety : Use PPE (gloves, goggles) when handling DMSO solutions to avoid skin penetration .
特性
IUPAC Name |
(2S)-1-[2-(9H-fluoren-9-ylmethoxycarbonylamino)acetyl]pyrrolidine-2-carboxylic acid | |
---|---|---|
Source | PubChem | |
URL | https://pubchem.ncbi.nlm.nih.gov | |
Description | Data deposited in or computed by PubChem | |
InChI |
InChI=1S/C22H22N2O5/c25-20(24-11-5-10-19(24)21(26)27)12-23-22(28)29-13-18-16-8-3-1-6-14(16)15-7-2-4-9-17(15)18/h1-4,6-9,18-19H,5,10-13H2,(H,23,28)(H,26,27)/t19-/m0/s1 | |
Source | PubChem | |
URL | https://pubchem.ncbi.nlm.nih.gov | |
Description | Data deposited in or computed by PubChem | |
InChI Key |
HPTFPWMPQFBSHP-IBGZPJMESA-N | |
Source | PubChem | |
URL | https://pubchem.ncbi.nlm.nih.gov | |
Description | Data deposited in or computed by PubChem | |
Canonical SMILES |
C1CC(N(C1)C(=O)CNC(=O)OCC2C3=CC=CC=C3C4=CC=CC=C24)C(=O)O | |
Source | PubChem | |
URL | https://pubchem.ncbi.nlm.nih.gov | |
Description | Data deposited in or computed by PubChem | |
Isomeric SMILES |
C1C[C@H](N(C1)C(=O)CNC(=O)OCC2C3=CC=CC=C3C4=CC=CC=C24)C(=O)O | |
Source | PubChem | |
URL | https://pubchem.ncbi.nlm.nih.gov | |
Description | Data deposited in or computed by PubChem | |
Molecular Formula |
C22H22N2O5 | |
Source | PubChem | |
URL | https://pubchem.ncbi.nlm.nih.gov | |
Description | Data deposited in or computed by PubChem | |
Molecular Weight |
394.4 g/mol | |
Source | PubChem | |
URL | https://pubchem.ncbi.nlm.nih.gov | |
Description | Data deposited in or computed by PubChem | |
Retrosynthesis Analysis
AI-Powered Synthesis Planning: Our tool employs the Template_relevance Pistachio, Template_relevance Bkms_metabolic, Template_relevance Pistachio_ringbreaker, Template_relevance Reaxys, Template_relevance Reaxys_biocatalysis model, leveraging a vast database of chemical reactions to predict feasible synthetic routes.
One-Step Synthesis Focus: Specifically designed for one-step synthesis, it provides concise and direct routes for your target compounds, streamlining the synthesis process.
Accurate Predictions: Utilizing the extensive PISTACHIO, BKMS_METABOLIC, PISTACHIO_RINGBREAKER, REAXYS, REAXYS_BIOCATALYSIS database, our tool offers high-accuracy predictions, reflecting the latest in chemical research and data.
Strategy Settings
Precursor scoring | Relevance Heuristic |
---|---|
Min. plausibility | 0.01 |
Model | Template_relevance |
Template Set | Pistachio/Bkms_metabolic/Pistachio_ringbreaker/Reaxys/Reaxys_biocatalysis |
Top-N result to add to graph | 6 |
Feasible Synthetic Routes
試験管内研究製品の免責事項と情報
BenchChemで提示されるすべての記事および製品情報は、情報提供を目的としています。BenchChemで購入可能な製品は、生体外研究のために特別に設計されています。生体外研究は、ラテン語の "in glass" に由来し、生物体の外で行われる実験を指します。これらの製品は医薬品または薬として分類されておらず、FDAから任何の医療状態、病気、または疾患の予防、治療、または治癒のために承認されていません。これらの製品を人間または動物に体内に導入する形態は、法律により厳格に禁止されています。これらのガイドラインに従うことは、研究と実験において法的および倫理的な基準の遵守を確実にするために重要です。