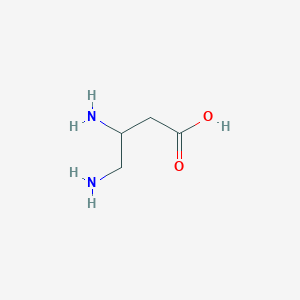
3,4-Diaminobutanoic acid
概要
説明
3,4-Diaminobutanoic acid, also known as DABA, is an amino acid that is not commonly found in proteins . It has a molecular weight of 191.06 and a molecular formula of C4H10N2O2 .
Synthesis Analysis
The synthesis of ®-3,4-Diaminobutanoic acid has been achieved through the desymmetrization of Dimethyl 3-(benzylamino)glutarate via enzymatic ammonolysis . Another chemoenzymatic approach has been used to synthesize a ®-3,4-diaminobutanoic acid derivative with suitable orthogonal protection for solid phase peptide synthesis .Molecular Structure Analysis
The molecular structure of 3,4-Diaminobutanoic acid is represented by the formula C4H10N2O2 . The average mass is 118.134 Da and the monoisotopic mass is 118.074226 Da .科学的研究の応用
Solid Phase Peptide Synthesis
The efficient chemoenzymatic synthesis of a ®-3,4-diaminobutanoic acid derivative has been achieved. The final compound has suitable orthogonal protection for solid phase peptide synthesis . This application is particularly useful in the field of biochemistry and molecular biology, where peptides are synthesized for various research purposes.
Cancer Treatment
3,4-Diaminobutanoic acid has been used in the development of peptide-drug conjugates for cancer treatment . These conjugates can act as carriers of chemotherapeutic agents and radionuclides by specifically targeting cancer cells. This application is significant in the field of oncology, where targeted therapies are being developed to improve the efficacy and reduce the side effects of cancer treatments.
Neurotoxin Quantification
3,4-Diaminobutanoic acid is a suitable reagent used in the quantification of the neurotoxin β-N-methylamino-L-alanine (BMAA) in seafood . This application is important in the field of food safety and neurology, as BMAA has been linked to various neurodegenerative diseases.
Peptide Therapeutics
Peptide therapeutics have played a significant role in different medical practices, including cancer treatment . 3,4-Diaminobutanoic acid, being an amino acid, can be used in the synthesis of these therapeutic peptides.
Drug Delivery
Modified peptides, which can include 3,4-Diaminobutanoic acid, have been developed as carriers for drugs . These peptide-drug conjugates have been evaluated in various phases of clinical trials for the treatment of different types of solid and leukemia cancer.
Immune System Regulation
Anticancer peptides (ACPs), which can be synthesized using 3,4-Diaminobutanoic acid, can regulate the immune system . This application is crucial in the field of immunology and cancer research, where the immune system’s role in cancer development and treatment is being extensively studied.
作用機序
Target of Action
3,4-Diaminobutanoic acid, also known as DABA, primarily targets GABA transaminase , an enzyme that converts GABA back to glutamate . This enzyme plays a crucial role in the regulation of GABA levels in the brain, which is an important inhibitory neurotransmitter.
Mode of Action
DABA acts as a non-competitive inhibitor of GABA transaminase . When the enzyme is inhibited, the conversion of GABA back to glutamate cannot occur, leading to elevated levels of GABA . Additionally, DABA has been observed to be a GABA reuptake inhibitor , which further elevates levels of GABA .
Biochemical Pathways
The primary biochemical pathway affected by DABA is the GABAergic pathway . By inhibiting GABA transaminase and acting as a GABA reuptake inhibitor, DABA increases the concentration of GABA in the synaptic cleft . This results in enhanced inhibitory neurotransmission, affecting various downstream neurological processes.
Pharmacokinetics
One study has shown that a daba-based compound exhibited high stability in mouse and human serum, with a half-life significantly greater than 24 hours . This suggests that DABA may have favorable ADME (Absorption, Distribution, Metabolism, and Excretion) properties, potentially leading to good bioavailability.
Result of Action
The primary result of DABA’s action is an increase in GABA levels, leading to enhanced inhibitory neurotransmission . This can have various effects on neurological function, potentially influencing mood, anxiety, and other aspects of brain function. It’s important to note that while daba has been shown to have anticonvulsant properties, over the long term, it could paradoxically cause convulsions .
Safety and Hazards
将来の方向性
The presence of β-amino acids like 3,4-Diaminobutanoic acid in natural products produces structural diversity and provides characteristic architectures beyond those of ordinary α-L-amino acids, thus generating significant and unique biological functions . The efficient chemoenzymatic synthesis of a ®-3,4-diaminobutanoic acid derivative has been achieved, which has suitable orthogonal protection for solid phase peptide synthesis . This suggests potential future directions in the field of peptide synthesis.
特性
IUPAC Name |
3,4-diaminobutanoic acid | |
---|---|---|
Source | PubChem | |
URL | https://pubchem.ncbi.nlm.nih.gov | |
Description | Data deposited in or computed by PubChem | |
InChI |
InChI=1S/C4H10N2O2/c5-2-3(6)1-4(7)8/h3H,1-2,5-6H2,(H,7,8) | |
Source | PubChem | |
URL | https://pubchem.ncbi.nlm.nih.gov | |
Description | Data deposited in or computed by PubChem | |
InChI Key |
XQKAYTBBWLDCBB-UHFFFAOYSA-N | |
Source | PubChem | |
URL | https://pubchem.ncbi.nlm.nih.gov | |
Description | Data deposited in or computed by PubChem | |
Canonical SMILES |
C(C(CN)N)C(=O)O | |
Source | PubChem | |
URL | https://pubchem.ncbi.nlm.nih.gov | |
Description | Data deposited in or computed by PubChem | |
Molecular Formula |
C4H10N2O2 | |
Source | PubChem | |
URL | https://pubchem.ncbi.nlm.nih.gov | |
Description | Data deposited in or computed by PubChem | |
DSSTOX Substance ID |
DTXSID10562684 | |
Record name | 3,4-Diaminobutanoic acid | |
Source | EPA DSSTox | |
URL | https://comptox.epa.gov/dashboard/DTXSID10562684 | |
Description | DSSTox provides a high quality public chemistry resource for supporting improved predictive toxicology. | |
Molecular Weight |
118.13 g/mol | |
Source | PubChem | |
URL | https://pubchem.ncbi.nlm.nih.gov | |
Description | Data deposited in or computed by PubChem | |
Product Name |
3,4-Diaminobutanoic acid | |
CAS RN |
131530-16-0 | |
Record name | 3,4-Diaminobutanoic acid | |
Source | EPA DSSTox | |
URL | https://comptox.epa.gov/dashboard/DTXSID10562684 | |
Description | DSSTox provides a high quality public chemistry resource for supporting improved predictive toxicology. | |
Retrosynthesis Analysis
AI-Powered Synthesis Planning: Our tool employs the Template_relevance Pistachio, Template_relevance Bkms_metabolic, Template_relevance Pistachio_ringbreaker, Template_relevance Reaxys, Template_relevance Reaxys_biocatalysis model, leveraging a vast database of chemical reactions to predict feasible synthetic routes.
One-Step Synthesis Focus: Specifically designed for one-step synthesis, it provides concise and direct routes for your target compounds, streamlining the synthesis process.
Accurate Predictions: Utilizing the extensive PISTACHIO, BKMS_METABOLIC, PISTACHIO_RINGBREAKER, REAXYS, REAXYS_BIOCATALYSIS database, our tool offers high-accuracy predictions, reflecting the latest in chemical research and data.
Strategy Settings
Precursor scoring | Relevance Heuristic |
---|---|
Min. plausibility | 0.01 |
Model | Template_relevance |
Template Set | Pistachio/Bkms_metabolic/Pistachio_ringbreaker/Reaxys/Reaxys_biocatalysis |
Top-N result to add to graph | 6 |
Feasible Synthetic Routes
Q & A
Q1: What are the different synthetic approaches for obtaining enantiomerically pure 3,4-diaminobutanoic acid?
A1: Two main synthetic strategies for enantiomerically pure 3,4-diaminobutanoic acid are highlighted in the provided research:
- Enzymatic Desymmetrization: Lipase B from Candida antarctica can efficiently catalyze the desymmetrization of dimethyl 3-(benzylamino)glutarate through ammonolysis. This method leads to the formation of enantiopure monoamides, which can be further converted to (R)-3,4-diaminobutanoic acid []. You can read more about this approach here: .
- Stereospecific Synthesis from D-Aspartic Acid: A practical and scalable method utilizes D-aspartic acid as a starting material for the stereospecific synthesis of (R)-3,4-diaminobutanoic acid dihydrochloride. This six-step synthesis proceeds with good overall yield and offers a potentially viable route for larger-scale production []. Further details on this method can be found here: .
Q2: Are there analytical techniques specifically designed for the detection and quantification of 3,4-diaminobutanoic acid?
A2: While the provided abstracts do not directly focus on analytical methods for 3,4-diaminobutanoic acid, a related paper describes a selective quantitation method for the neurotoxin β-Methylamino-L-alanine (BMAA) using hydrophilic-interaction liquid chromatography–differential mobility spectrometry–tandem mass spectrometry (HILIC–DMS–MS/MS) []. This suggests that similar chromatographic and mass spectrometry techniques could potentially be adapted for the analysis of 3,4-diaminobutanoic acid.
試験管内研究製品の免責事項と情報
BenchChemで提示されるすべての記事および製品情報は、情報提供を目的としています。BenchChemで購入可能な製品は、生体外研究のために特別に設計されています。生体外研究は、ラテン語の "in glass" に由来し、生物体の外で行われる実験を指します。これらの製品は医薬品または薬として分類されておらず、FDAから任何の医療状態、病気、または疾患の予防、治療、または治癒のために承認されていません。これらの製品を人間または動物に体内に導入する形態は、法律により厳格に禁止されています。これらのガイドラインに従うことは、研究と実験において法的および倫理的な基準の遵守を確実にするために重要です。