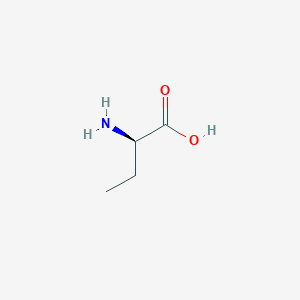
D-2-アミノ酪酸
概要
説明
D-2-Aminobutyric acid is a non-proteinogenic amino acid with the molecular formula C4H9NO2. It is an enantiomer of 2-aminobutyric acid and is characterized by its chiral center, which gives it specific optical activity. This compound is of significant interest due to its applications in various fields, including pharmaceuticals, agriculture, and food industries .
科学的研究の応用
D-2-Aminobutyric acid has a wide range of applications in scientific research:
Chemistry: It is used as a building block in the synthesis of complex molecules and as a chiral auxiliary in asymmetric synthesis.
Biology: It serves as a substrate for studying enzyme kinetics and mechanisms.
Medicine: It is used in the synthesis of pharmaceuticals, including antibiotics and enzyme inhibitors.
Industry: It is employed in the production of agrochemicals and food additives.
作用機序
Target of Action
D-2-Aminobutyric acid primarily targets the γ-Aminobutyric acid sub-type A receptors (GABA A Rs), which are the most prominent inhibitory neurotransmitter receptors in the central nervous system (CNS) . These receptors are a family of ligand-gated ion channels with significant physiological and therapeutic implications .
Mode of Action
D-2-Aminobutyric acid interacts with its targets by binding to post-synaptic GABA receptors (GABA A and GABA B), which modulate ion channels, hyperpolarize the cell, and prevent action potential transmission . This interaction results in elevated levels of GABA .
Biochemical Pathways
D-2-Aminobutyric acid affects the GABAergic system, which is critical in neurodevelopmental disorders . It is involved in the synthesis of antibiotics, antiproliferatives, and inhibitors of angiotensin . A multi-enzymatic system made of L-threonine ammonia lyase from E. coli, DAADH, and formate dehydrogenase (from Pseudomonas sp. 101 or Candida boidinii) was set up to produce D-2-aminobutyric acid using L-threonine .
Pharmacokinetics
For instance, the GABA receptor potentiator HSK3486, which also targets GABA receptors, has been studied for its pharmacokinetic properties . Similar studies could provide insights into the pharmacokinetics of D-2-Aminobutyric acid.
Result of Action
The result of D-2-Aminobutyric acid’s action is the elevation of GABA levels . This can have a significant effect on normal brain function . Dysfunction of the GABAergic system contributes to the development of several diseases .
生化学分析
Biochemical Properties
D-2-Aminobutyric acid is synthesized from L-threonine by L-threonine ammonia lyase, which is then converted to D-2-Aminobutyric acid by D-amino acid dehydrogenase . The enzyme formate dehydrogenase is also involved in this process .
Cellular Effects
D-2-Aminobutyric acid is involved in several physiological processes. It has been found to have a role in autoimmune diseases like multiple sclerosis, type 1 diabetes, and rheumatoid arthritis and may modulate the immune response to infections .
Molecular Mechanism
The molecular mechanism of D-2-Aminobutyric acid involves its interaction with various enzymes and proteins. For instance, it interacts with L-threonine ammonia lyase, D-amino acid dehydrogenase, and formate dehydrogenase during its synthesis .
Temporal Effects in Laboratory Settings
In laboratory settings, D-2-Aminobutyric acid has been shown to be produced with high yield and enantioselective excess . This indicates that the compound is stable and does not degrade significantly over time.
Dosage Effects in Animal Models
Given its role in modulating immune responses, it is likely that its effects would vary depending on the dosage .
Metabolic Pathways
D-2-Aminobutyric acid is involved in the metabolic pathway that converts L-threonine to D-2-Aminobutyric acid. This pathway involves the enzymes L-threonine ammonia lyase, D-amino acid dehydrogenase, and formate dehydrogenase .
Subcellular Localization
The subcellular localization of D-2-Aminobutyric acid is also not well known. Given that it is synthesized from L-threonine, it is likely that it is found in the same subcellular compartments where this metabolic process occurs .
準備方法
Synthetic Routes and Reaction Conditions: D-2-Aminobutyric acid can be synthesized through several methods. One common approach involves the enzymatic synthesis using a multi-enzymatic system. This system typically includes L-threonine ammonia lyase, D-amino acid dehydrogenase, and formate dehydrogenase. The process starts with L-threonine, which undergoes deamination to form 2-ketobutyrate. This intermediate is then reductively aminated to produce D-2-Aminobutyric acid with high optical purity .
Industrial Production Methods: In industrial settings, D-2-Aminobutyric acid is often produced using biocatalytic processes due to their efficiency and environmental benefits. The use of recombinant microorganisms engineered to express the necessary enzymes allows for large-scale production. This method is preferred for its high yield and enantioselectivity .
化学反応の分析
Types of Reactions: D-2-Aminobutyric acid undergoes various chemical reactions, including:
Oxidation: It can be oxidized to form corresponding keto acids.
Reduction: It can be reduced to form amines.
Substitution: It can participate in substitution reactions to form derivatives.
Common Reagents and Conditions:
Oxidation: Common oxidizing agents include potassium permanganate and hydrogen peroxide.
Reduction: Reducing agents such as sodium borohydride and lithium aluminum hydride are often used.
Substitution: Reagents like alkyl halides and acyl chlorides are used under basic or acidic conditions.
Major Products: The major products formed from these reactions include keto acids, amines, and various substituted derivatives .
類似化合物との比較
- L-2-Aminobutyric acid
- DL-2-Aminobutyric acid
- 2-Aminoisobutyric acid
Comparison: D-2-Aminobutyric acid is unique due to its specific chiral configuration, which imparts distinct biological activity compared to its L-enantiomer. Unlike DL-2-Aminobutyric acid, which is a racemic mixture, D-2-Aminobutyric acid is optically pure and exhibits higher specificity in biochemical reactions. 2-Aminoisobutyric acid, on the other hand, has a different structural configuration, leading to varied reactivity and applications .
特性
IUPAC Name |
(2R)-2-aminobutanoic acid | |
---|---|---|
Source | PubChem | |
URL | https://pubchem.ncbi.nlm.nih.gov | |
Description | Data deposited in or computed by PubChem | |
InChI |
InChI=1S/C4H9NO2/c1-2-3(5)4(6)7/h3H,2,5H2,1H3,(H,6,7)/t3-/m1/s1 | |
Source | PubChem | |
URL | https://pubchem.ncbi.nlm.nih.gov | |
Description | Data deposited in or computed by PubChem | |
InChI Key |
QWCKQJZIFLGMSD-GSVOUGTGSA-N | |
Source | PubChem | |
URL | https://pubchem.ncbi.nlm.nih.gov | |
Description | Data deposited in or computed by PubChem | |
Canonical SMILES |
CCC(C(=O)O)N | |
Source | PubChem | |
URL | https://pubchem.ncbi.nlm.nih.gov | |
Description | Data deposited in or computed by PubChem | |
Isomeric SMILES |
CC[C@H](C(=O)O)N | |
Source | PubChem | |
URL | https://pubchem.ncbi.nlm.nih.gov | |
Description | Data deposited in or computed by PubChem | |
Molecular Formula |
C4H9NO2 | |
Source | PubChem | |
URL | https://pubchem.ncbi.nlm.nih.gov | |
Description | Data deposited in or computed by PubChem | |
DSSTOX Substance ID |
DTXSID701314996 | |
Record name | D-α-Aminobutyric acid | |
Source | EPA DSSTox | |
URL | https://comptox.epa.gov/dashboard/DTXSID701314996 | |
Description | DSSTox provides a high quality public chemistry resource for supporting improved predictive toxicology. | |
Molecular Weight |
103.12 g/mol | |
Source | PubChem | |
URL | https://pubchem.ncbi.nlm.nih.gov | |
Description | Data deposited in or computed by PubChem | |
Physical Description |
Solid | |
Record name | D-alpha-Aminobutyric acid | |
Source | Human Metabolome Database (HMDB) | |
URL | http://www.hmdb.ca/metabolites/HMDB0000650 | |
Description | The Human Metabolome Database (HMDB) is a freely available electronic database containing detailed information about small molecule metabolites found in the human body. | |
Explanation | HMDB is offered to the public as a freely available resource. Use and re-distribution of the data, in whole or in part, for commercial purposes requires explicit permission of the authors and explicit acknowledgment of the source material (HMDB) and the original publication (see the HMDB citing page). We ask that users who download significant portions of the database cite the HMDB paper in any resulting publications. | |
Solubility |
278 mg/mL | |
Record name | D-alpha-Aminobutyric acid | |
Source | Human Metabolome Database (HMDB) | |
URL | http://www.hmdb.ca/metabolites/HMDB0000650 | |
Description | The Human Metabolome Database (HMDB) is a freely available electronic database containing detailed information about small molecule metabolites found in the human body. | |
Explanation | HMDB is offered to the public as a freely available resource. Use and re-distribution of the data, in whole or in part, for commercial purposes requires explicit permission of the authors and explicit acknowledgment of the source material (HMDB) and the original publication (see the HMDB citing page). We ask that users who download significant portions of the database cite the HMDB paper in any resulting publications. | |
CAS No. |
2623-91-8 | |
Record name | D-α-Aminobutyric acid | |
Source | CAS Common Chemistry | |
URL | https://commonchemistry.cas.org/detail?cas_rn=2623-91-8 | |
Description | CAS Common Chemistry is an open community resource for accessing chemical information. Nearly 500,000 chemical substances from CAS REGISTRY cover areas of community interest, including common and frequently regulated chemicals, and those relevant to high school and undergraduate chemistry classes. This chemical information, curated by our expert scientists, is provided in alignment with our mission as a division of the American Chemical Society. | |
Explanation | The data from CAS Common Chemistry is provided under a CC-BY-NC 4.0 license, unless otherwise stated. | |
Record name | D-alpha-Aminobutyric acid | |
Source | ChemIDplus | |
URL | https://pubchem.ncbi.nlm.nih.gov/substance/?source=chemidplus&sourceid=0002623918 | |
Description | ChemIDplus is a free, web search system that provides access to the structure and nomenclature authority files used for the identification of chemical substances cited in National Library of Medicine (NLM) databases, including the TOXNET system. | |
Record name | D-α-Aminobutyric acid | |
Source | EPA DSSTox | |
URL | https://comptox.epa.gov/dashboard/DTXSID701314996 | |
Description | DSSTox provides a high quality public chemistry resource for supporting improved predictive toxicology. | |
Record name | D-(-)-2-aminobutyric acid | |
Source | European Chemicals Agency (ECHA) | |
URL | https://echa.europa.eu/substance-information/-/substanceinfo/100.018.260 | |
Description | The European Chemicals Agency (ECHA) is an agency of the European Union which is the driving force among regulatory authorities in implementing the EU's groundbreaking chemicals legislation for the benefit of human health and the environment as well as for innovation and competitiveness. | |
Explanation | Use of the information, documents and data from the ECHA website is subject to the terms and conditions of this Legal Notice, and subject to other binding limitations provided for under applicable law, the information, documents and data made available on the ECHA website may be reproduced, distributed and/or used, totally or in part, for non-commercial purposes provided that ECHA is acknowledged as the source: "Source: European Chemicals Agency, http://echa.europa.eu/". Such acknowledgement must be included in each copy of the material. ECHA permits and encourages organisations and individuals to create links to the ECHA website under the following cumulative conditions: Links can only be made to webpages that provide a link to the Legal Notice page. | |
Record name | D-alpha-Aminobutyric acid | |
Source | Human Metabolome Database (HMDB) | |
URL | http://www.hmdb.ca/metabolites/HMDB0000650 | |
Description | The Human Metabolome Database (HMDB) is a freely available electronic database containing detailed information about small molecule metabolites found in the human body. | |
Explanation | HMDB is offered to the public as a freely available resource. Use and re-distribution of the data, in whole or in part, for commercial purposes requires explicit permission of the authors and explicit acknowledgment of the source material (HMDB) and the original publication (see the HMDB citing page). We ask that users who download significant portions of the database cite the HMDB paper in any resulting publications. | |
Retrosynthesis Analysis
AI-Powered Synthesis Planning: Our tool employs the Template_relevance Pistachio, Template_relevance Bkms_metabolic, Template_relevance Pistachio_ringbreaker, Template_relevance Reaxys, Template_relevance Reaxys_biocatalysis model, leveraging a vast database of chemical reactions to predict feasible synthetic routes.
One-Step Synthesis Focus: Specifically designed for one-step synthesis, it provides concise and direct routes for your target compounds, streamlining the synthesis process.
Accurate Predictions: Utilizing the extensive PISTACHIO, BKMS_METABOLIC, PISTACHIO_RINGBREAKER, REAXYS, REAXYS_BIOCATALYSIS database, our tool offers high-accuracy predictions, reflecting the latest in chemical research and data.
Strategy Settings
Precursor scoring | Relevance Heuristic |
---|---|
Min. plausibility | 0.01 |
Model | Template_relevance |
Template Set | Pistachio/Bkms_metabolic/Pistachio_ringbreaker/Reaxys/Reaxys_biocatalysis |
Top-N result to add to graph | 6 |
Feasible Synthetic Routes
Q1: What is known about the conformational preferences of D-2-Aminobutyric acid when incorporated into larger peptides?
A1: Studies focusing on the dipeptide taste ligand, L‐aspartyl‐D‐2‐aminobutyric acid‐(S)‐α‐ethylbenzylamide, reveal that D-2-Aminobutyric acid can adopt different side-chain conformations (g−, g+, and t) when part of an N-substituted aspartyl residue. This flexibility was observed in X-ray crystallographic studies of the dipeptide, which showed six distinct conformers within the unit cell. Despite these conformational variations, the dipeptide retained its sweet taste properties. [, ]
Q2: How does the structure of D-2-Aminobutyric acid relate to its ability to form co-crystals with other amino acids?
A2: D-2-Aminobutyric acid readily forms co-crystals with various L-amino acids, including L-Norvaline and L-Phenylalanine. These complexes typically exhibit a 1:1 stoichiometry and display hydrogen-bonding patterns characteristic of LD–LD interactions, forming 2D hydrogen-bonded bilayers. [, ] The linear side chain of D-2-Aminobutyric acid appears to contribute to the formation of these specific packing arrangements.
Q3: Can D-2-Aminobutyric acid be used in the synthesis of cyclosporin analogues?
A3: Yes, cell-free enzymatic systems derived from the fungus Beauveria nivea can incorporate D-2-Aminobutyric acid into the cyclosporin structure. This has enabled the production of [D-2-Aminobutyric acid]Cyclosporin A, a novel analogue. [] The immunosuppressive activity of this and other synthesized analogues requires further investigation.
Q4: What is the role of D-2-Aminobutyric acid in the structure-activity relationship of the antitumor bicyclic hexapeptide RA-VII?
A4: While not naturally present in RA-VII, D-2-Aminobutyric acid has been used as a replacement for D-Alanine at position 1 in synthetic analogues. Studies indicated that substituting D-Alanine with D-2-Aminobutyric acid, or other larger amino acids, at position 1 decreased the cytotoxic activity of RA-VII analogues. This suggests that the size of the side chain at this position is crucial for maintaining the bioactive conformation and thus, the antitumor activity. [, ]
Q5: Are there efficient methods for synthesizing D-2-Aminobutyric acid with high optical purity?
A5: Yes, a highly atom-economic tri-enzymatic catalytic system has been developed for the synthesis of D-2-Aminobutyric acid using L-Threonine as the starting material. This system, comprised of L-Threonine ammonia lyase (L-TAL), D-Amino acid dehydrogenase (D-AADH), and formate dehydrogenase (FDH), produces D-2-Aminobutyric acid with >90% yield and >99% enantiomeric excess. []
Q6: What are the implications of D-amino acid degradation pathways in E. coli for the biotechnological production of D-amino acids?
A6: E. coli, a commonly used host for biocatalytic production, expresses enzymes like D-Amino acid dehydrogenase (DadA) and D-Serine dehydratase (DsdA) that degrade D-amino acids. Deletion of the dadA gene significantly reduced degradation of D-Methionine, D-Phenylalanine, and almost completely abolished D-2-Aminobutyric acid degradation. Further deletion of dsdA alongside dadA nearly eliminated D-Serine and D-Phenylalanine degradation. These findings suggest that dadA and dsdA deletion mutants are promising hosts for enhanced biocatalytic production of D-amino acids, including D-2-Aminobutyric acid, using systems like the hydantoinase pathway. []
Q7: Has D-2-Aminobutyric acid been explored for its potential in nonlinear optical applications?
A7: Recent research suggests that the D-2-Aminobutyric acid L-Norvaline co-crystal exhibits promising third-order nonlinear optical (NLO) properties. While still in early stages, this research highlights the potential of this co-crystal for applications in optoelectronics and photonics. []
試験管内研究製品の免責事項と情報
BenchChemで提示されるすべての記事および製品情報は、情報提供を目的としています。BenchChemで購入可能な製品は、生体外研究のために特別に設計されています。生体外研究は、ラテン語の "in glass" に由来し、生物体の外で行われる実験を指します。これらの製品は医薬品または薬として分類されておらず、FDAから任何の医療状態、病気、または疾患の予防、治療、または治癒のために承認されていません。これらの製品を人間または動物に体内に導入する形態は、法律により厳格に禁止されています。これらのガイドラインに従うことは、研究と実験において法的および倫理的な基準の遵守を確実にするために重要です。