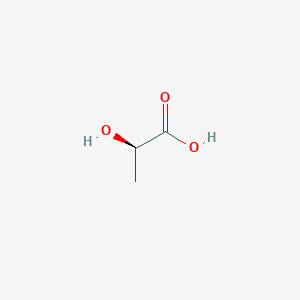
D-Lactic acid
概要
説明
D-乳酸は、分子式がC3H6O3である有機化合物です。乳酸の2つの光学異性体の1つであり、もう1つはL-乳酸です。D-乳酸は、特定の種類の微生物によって生成され、一部の代謝経路にも見られます。これはキラル分子であり、重ね合わせることができない鏡像があります。 D-乳酸は、L-乳酸と比較してヒトの代謝ではあまり一般的ではありませんが、産業上および科学的に重要な用途があります .
準備方法
合成ルートと反応条件: D-乳酸は、化学的に合成することも、微生物発酵によって生成することもできます。化学合成には、アセトアルデヒドとシアン化水素の反応によるラクトニトリルの生成が含まれ、ラクトニトリルは加水分解されて乳酸を生成します。 この方法は通常、D-乳酸とL-乳酸のラセミ混合物を生成します .
工業生産方法: D-乳酸の工業生産は、主に微生物発酵に依存しています。ラクトバチルスデルブルエッキなどの特定の種類の細菌を使用して、グルコースやラクトースなどの炭水化物を発酵させます。発酵プロセスは、光学的に純粋なD-乳酸の生成を保証するために、制御された条件下で行われます。 次に、発酵ブロスを、沈殿、溶媒抽出、膜分離などのさまざまな精製プロセスにかけて、純粋なD-乳酸を得ます .
化学反応の分析
反応の種類: D-乳酸は、酸化、還元、エステル化など、いくつかの種類の化学反応を起こします。ピルビン酸に酸化され、プロピオン酸に還元されます。 D-乳酸をアルコールでエステル化すると、ラクトエステルが生成されます .
一般的な試薬と条件: D-乳酸の反応で使用される一般的な試薬には、過マンガン酸カリウムなどの酸化剤と、水素化ホウ素ナトリウムなどの還元剤が含まれます。 エステル化反応には、通常、アルコールと硫酸などの酸触媒が使用されます .
生成される主な生成物: D-乳酸の反応から生成される主な生成物には、ピルビン酸、プロピオン酸、およびさまざまなラクトエステルが含まれます。 これらの生成物は、化学および製薬産業でさまざまな用途があります .
科学研究の用途
D-乳酸は、幅広い科学研究の用途があります。化学では、エナンチオマー的に純粋な化合物を合成するためのキラルビルディングブロックとして使用されます。生物学では、特定の代謝異常のマーカーとして使用され、微生物代謝の研究で使用されます。医学では、D-乳酸は、薬物送達システムや組織工学のための生分解性ポリマーの製造に使用されます。 産業的には、接着剤、プラスチックの製造に使用され、食品の防腐剤として使用されます .
科学的研究の応用
D-lactic acid has a wide range of scientific research applications. In chemistry, it is used as a chiral building block for the synthesis of enantiomerically pure compounds. In biology, it serves as a marker for certain metabolic disorders and is used in studies of microbial metabolism. In medicine, this compound is used in the production of biodegradable polymers for drug delivery systems and tissue engineering. Industrially, it is used in the production of adhesives, plastics, and as a preservative in food products .
作用機序
D-乳酸は、さまざまな分子標的と経路を通じてその効果を発揮します。体内ではピルビン酸に代謝され、ピルビン酸はエネルギー産生のためにクエン酸回路に入ります。微生物発酵では、D-乳酸は、乳酸脱水素酵素を介してピルビン酸から生成されます。 D-乳酸の蓄積は、代謝性アシドーシスにつながる可能性があります。代謝性アシドーシスは、血液の酸性度が上昇する状態です .
類似化合物との比較
D-乳酸はL-乳酸に似ていますが、光学活性と代謝経路が異なります。L-乳酸はヒトの代謝で一般的に生成されますが、D-乳酸は特定の種類の微生物によって生成されます。その他の類似化合物には、グリコール酸、酢酸、プロピオン酸などがあります。 D-乳酸は、生分解性ポリマーを形成する能力とそのキラル合成における特定の用途がユニークです .
生物活性
D-lactic acid, an enantiomer of lactic acid, is produced primarily by certain bacteria in the human gut and has significant biological activity, particularly in relation to metabolic disorders, gastrointestinal health, and neurotoxicity. This article synthesizes current research findings on the biological activity of this compound, highlighting its implications in various health conditions.
This compound (C₃H₆O₃) is a three-carbon carboxylic acid that can be produced through the fermentation of carbohydrates by specific strains of bacteria, such as Lactobacillus and Leuconostoc. Unlike its L-isomer, this compound is not a standard metabolite in human metabolism and is primarily generated in pathological states, such as short bowel syndrome (SBS) and bacterial overgrowth.
D-Lactic Acidosis
D-lactic acidosis (DLA) is a metabolic condition characterized by elevated levels of this compound in the bloodstream, often resulting from malabsorption of carbohydrates. In pediatric patients with SBS, DLA can lead to severe neurological symptoms due to the neurotoxic effects of D-lactate accumulation. For instance, studies have shown that children with SBS can exhibit serum D-lactate concentrations exceeding 0.25 mmol/L, correlating with metabolic acidosis and neurological manifestations such as confusion and ataxia .
Table 1: Case Studies on D-Lactic Acidosis
Study | Patient Group | Serum D-Lactate Levels (mmol/L) | Symptoms |
---|---|---|---|
Yang et al. | IBD Patients | 7.18 ± 0.77 (before treatment) | Metabolic acidosis |
Cai et al. | Active Crohn's Disease | 0.18 ± 0.05 | Neurological symptoms |
Demircan et al. | Perforated Appendicitis | 0.44 ± 0.08 | Metabolic disturbances |
Neurotoxic Effects
The neurotoxic effects of this compound are particularly concerning. Research indicates that high levels of D-lactate can cause confusion, dizziness, and aggressive behavior independent of blood pH changes . In animal models, such as Holstein calves, the presence of D-lactate has been linked to ataxic gait and encephalopathy .
This compound's neurotoxicity may be attributed to its ability to disrupt normal neuronal function and induce oxidative stress. The compound has been shown to affect neurotransmitter systems and may contribute to inflammatory responses in the central nervous system .
Diagnostic Applications
This compound serves as a potential biomarker for various gastrointestinal disorders. Elevated levels are often indicative of dysbiosis or intestinal permeability issues. Recent studies have demonstrated that measuring D-lactate can aid in diagnosing conditions like sepsis associated with acute gastrointestinal injury (AGI). The area under the curve (AUC) for this compound as a diagnostic marker was found to be 0.881, outperforming other biomarkers like fecal calprotectin .
Research Findings and Future Directions
Recent literature emphasizes the need for further research into the enzymatic pathways involving this compound metabolism and its implications for human health:
- Metabolic Pathways : The primary enzymes involved in D-lactate metabolism include glyoxalase III, which catalyzes the conversion of D-lactate to methylglyoxal, suggesting a detoxification route .
- Clinical Implications : Understanding the role of D-lactate in diseases beyond SBS could unveil new therapeutic targets for managing metabolic acidosis and neurotoxicity.
特性
IUPAC Name |
(2R)-2-hydroxypropanoic acid | |
---|---|---|
Source | PubChem | |
URL | https://pubchem.ncbi.nlm.nih.gov | |
Description | Data deposited in or computed by PubChem | |
InChI |
InChI=1S/C3H6O3/c1-2(4)3(5)6/h2,4H,1H3,(H,5,6)/t2-/m1/s1 | |
Source | PubChem | |
URL | https://pubchem.ncbi.nlm.nih.gov | |
Description | Data deposited in or computed by PubChem | |
InChI Key |
JVTAAEKCZFNVCJ-UWTATZPHSA-N | |
Source | PubChem | |
URL | https://pubchem.ncbi.nlm.nih.gov | |
Description | Data deposited in or computed by PubChem | |
Canonical SMILES |
CC(C(=O)O)O | |
Source | PubChem | |
URL | https://pubchem.ncbi.nlm.nih.gov | |
Description | Data deposited in or computed by PubChem | |
Isomeric SMILES |
C[C@H](C(=O)O)O | |
Source | PubChem | |
URL | https://pubchem.ncbi.nlm.nih.gov | |
Description | Data deposited in or computed by PubChem | |
Molecular Formula |
C3H6O3 | |
Source | PubChem | |
URL | https://pubchem.ncbi.nlm.nih.gov | |
Description | Data deposited in or computed by PubChem | |
Related CAS |
106989-11-1 | |
Record name | Poly[(+)-lactic acid] | |
Source | CAS Common Chemistry | |
URL | https://commonchemistry.cas.org/detail?cas_rn=106989-11-1 | |
Description | CAS Common Chemistry is an open community resource for accessing chemical information. Nearly 500,000 chemical substances from CAS REGISTRY cover areas of community interest, including common and frequently regulated chemicals, and those relevant to high school and undergraduate chemistry classes. This chemical information, curated by our expert scientists, is provided in alignment with our mission as a division of the American Chemical Society. | |
Explanation | The data from CAS Common Chemistry is provided under a CC-BY-NC 4.0 license, unless otherwise stated. | |
DSSTOX Substance ID |
DTXSID0047030 | |
Record name | D-Lactic acid | |
Source | EPA DSSTox | |
URL | https://comptox.epa.gov/dashboard/DTXSID0047030 | |
Description | DSSTox provides a high quality public chemistry resource for supporting improved predictive toxicology. | |
Molecular Weight |
90.08 g/mol | |
Source | PubChem | |
URL | https://pubchem.ncbi.nlm.nih.gov | |
Description | Data deposited in or computed by PubChem | |
Physical Description |
Solid | |
Record name | D-Lactic acid | |
Source | Human Metabolome Database (HMDB) | |
URL | http://www.hmdb.ca/metabolites/HMDB0001311 | |
Description | The Human Metabolome Database (HMDB) is a freely available electronic database containing detailed information about small molecule metabolites found in the human body. | |
Explanation | HMDB is offered to the public as a freely available resource. Use and re-distribution of the data, in whole or in part, for commercial purposes requires explicit permission of the authors and explicit acknowledgment of the source material (HMDB) and the original publication (see the HMDB citing page). We ask that users who download significant portions of the database cite the HMDB paper in any resulting publications. | |
CAS No. |
10326-41-7 | |
Record name | D-Lactic acid | |
Source | CAS Common Chemistry | |
URL | https://commonchemistry.cas.org/detail?cas_rn=10326-41-7 | |
Description | CAS Common Chemistry is an open community resource for accessing chemical information. Nearly 500,000 chemical substances from CAS REGISTRY cover areas of community interest, including common and frequently regulated chemicals, and those relevant to high school and undergraduate chemistry classes. This chemical information, curated by our expert scientists, is provided in alignment with our mission as a division of the American Chemical Society. | |
Explanation | The data from CAS Common Chemistry is provided under a CC-BY-NC 4.0 license, unless otherwise stated. | |
Record name | D-Lactic acid | |
Source | ChemIDplus | |
URL | https://pubchem.ncbi.nlm.nih.gov/substance/?source=chemidplus&sourceid=0010326417 | |
Description | ChemIDplus is a free, web search system that provides access to the structure and nomenclature authority files used for the identification of chemical substances cited in National Library of Medicine (NLM) databases, including the TOXNET system. | |
Record name | D-Lactic acid | |
Source | DrugBank | |
URL | https://www.drugbank.ca/drugs/DB03066 | |
Description | The DrugBank database is a unique bioinformatics and cheminformatics resource that combines detailed drug (i.e. chemical, pharmacological and pharmaceutical) data with comprehensive drug target (i.e. sequence, structure, and pathway) information. | |
Explanation | Creative Common's Attribution-NonCommercial 4.0 International License (http://creativecommons.org/licenses/by-nc/4.0/legalcode) | |
Record name | Propanoic acid, 2-hydroxy-, (2R)- | |
Source | EPA Chemicals under the TSCA | |
URL | https://www.epa.gov/chemicals-under-tsca | |
Description | EPA Chemicals under the Toxic Substances Control Act (TSCA) collection contains information on chemicals and their regulations under TSCA, including non-confidential content from the TSCA Chemical Substance Inventory and Chemical Data Reporting. | |
Record name | D-Lactic acid | |
Source | EPA DSSTox | |
URL | https://comptox.epa.gov/dashboard/DTXSID0047030 | |
Description | DSSTox provides a high quality public chemistry resource for supporting improved predictive toxicology. | |
Record name | (R)-lactic acid | |
Source | European Chemicals Agency (ECHA) | |
URL | https://echa.europa.eu/substance-information/-/substanceinfo/100.030.636 | |
Description | The European Chemicals Agency (ECHA) is an agency of the European Union which is the driving force among regulatory authorities in implementing the EU's groundbreaking chemicals legislation for the benefit of human health and the environment as well as for innovation and competitiveness. | |
Explanation | Use of the information, documents and data from the ECHA website is subject to the terms and conditions of this Legal Notice, and subject to other binding limitations provided for under applicable law, the information, documents and data made available on the ECHA website may be reproduced, distributed and/or used, totally or in part, for non-commercial purposes provided that ECHA is acknowledged as the source: "Source: European Chemicals Agency, http://echa.europa.eu/". Such acknowledgement must be included in each copy of the material. ECHA permits and encourages organisations and individuals to create links to the ECHA website under the following cumulative conditions: Links can only be made to webpages that provide a link to the Legal Notice page. | |
Record name | LACTIC ACID, D- | |
Source | FDA Global Substance Registration System (GSRS) | |
URL | https://gsrs.ncats.nih.gov/ginas/app/beta/substances/3Q6M5SET7W | |
Description | The FDA Global Substance Registration System (GSRS) enables the efficient and accurate exchange of information on what substances are in regulated products. Instead of relying on names, which vary across regulatory domains, countries, and regions, the GSRS knowledge base makes it possible for substances to be defined by standardized, scientific descriptions. | |
Explanation | Unless otherwise noted, the contents of the FDA website (www.fda.gov), both text and graphics, are not copyrighted. They are in the public domain and may be republished, reprinted and otherwise used freely by anyone without the need to obtain permission from FDA. Credit to the U.S. Food and Drug Administration as the source is appreciated but not required. | |
Record name | D-Lactic acid | |
Source | Human Metabolome Database (HMDB) | |
URL | http://www.hmdb.ca/metabolites/HMDB0001311 | |
Description | The Human Metabolome Database (HMDB) is a freely available electronic database containing detailed information about small molecule metabolites found in the human body. | |
Explanation | HMDB is offered to the public as a freely available resource. Use and re-distribution of the data, in whole or in part, for commercial purposes requires explicit permission of the authors and explicit acknowledgment of the source material (HMDB) and the original publication (see the HMDB citing page). We ask that users who download significant portions of the database cite the HMDB paper in any resulting publications. | |
Melting Point |
52.8 °C | |
Record name | D-Lactic acid | |
Source | DrugBank | |
URL | https://www.drugbank.ca/drugs/DB03066 | |
Description | The DrugBank database is a unique bioinformatics and cheminformatics resource that combines detailed drug (i.e. chemical, pharmacological and pharmaceutical) data with comprehensive drug target (i.e. sequence, structure, and pathway) information. | |
Explanation | Creative Common's Attribution-NonCommercial 4.0 International License (http://creativecommons.org/licenses/by-nc/4.0/legalcode) | |
Record name | D-Lactic acid | |
Source | Human Metabolome Database (HMDB) | |
URL | http://www.hmdb.ca/metabolites/HMDB0001311 | |
Description | The Human Metabolome Database (HMDB) is a freely available electronic database containing detailed information about small molecule metabolites found in the human body. | |
Explanation | HMDB is offered to the public as a freely available resource. Use and re-distribution of the data, in whole or in part, for commercial purposes requires explicit permission of the authors and explicit acknowledgment of the source material (HMDB) and the original publication (see the HMDB citing page). We ask that users who download significant portions of the database cite the HMDB paper in any resulting publications. | |
Synthesis routes and methods I
Procedure details
Synthesis routes and methods II
Procedure details
Synthesis routes and methods III
Procedure details
Synthesis routes and methods IV
Procedure details
Synthesis routes and methods V
Procedure details
Retrosynthesis Analysis
AI-Powered Synthesis Planning: Our tool employs the Template_relevance Pistachio, Template_relevance Bkms_metabolic, Template_relevance Pistachio_ringbreaker, Template_relevance Reaxys, Template_relevance Reaxys_biocatalysis model, leveraging a vast database of chemical reactions to predict feasible synthetic routes.
One-Step Synthesis Focus: Specifically designed for one-step synthesis, it provides concise and direct routes for your target compounds, streamlining the synthesis process.
Accurate Predictions: Utilizing the extensive PISTACHIO, BKMS_METABOLIC, PISTACHIO_RINGBREAKER, REAXYS, REAXYS_BIOCATALYSIS database, our tool offers high-accuracy predictions, reflecting the latest in chemical research and data.
Strategy Settings
Precursor scoring | Relevance Heuristic |
---|---|
Min. plausibility | 0.01 |
Model | Template_relevance |
Template Set | Pistachio/Bkms_metabolic/Pistachio_ringbreaker/Reaxys/Reaxys_biocatalysis |
Top-N result to add to graph | 6 |
Feasible Synthetic Routes
試験管内研究製品の免責事項と情報
BenchChemで提示されるすべての記事および製品情報は、情報提供を目的としています。BenchChemで購入可能な製品は、生体外研究のために特別に設計されています。生体外研究は、ラテン語の "in glass" に由来し、生物体の外で行われる実験を指します。これらの製品は医薬品または薬として分類されておらず、FDAから任何の医療状態、病気、または疾患の予防、治療、または治癒のために承認されていません。これらの製品を人間または動物に体内に導入する形態は、法律により厳格に禁止されています。これらのガイドラインに従うことは、研究と実験において法的および倫理的な基準の遵守を確実にするために重要です。