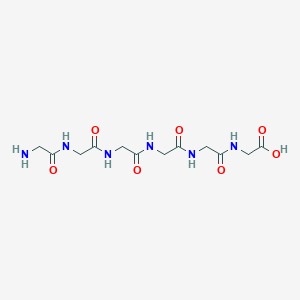
Hexaglycine
概要
説明
グリシンは最も単純なアミノ酸であり、グリシン6中のその繰り返し配列は、分子式C12H20N6O7、分子量360.32 g/molの分子をもたらします 。グリシン6は主に研究目的で使用されており、さまざまな生化学的および生物物理学的研究における役割で知られています。
準備方法
合成経路と反応条件: グリシン6は、標準的な固相ペプチド合成(SPPS)技術を使用して合成できます。このプロセスには、固体樹脂に固定された成長中のペプチド鎖へのグリシン残基の逐次添加が含まれます。合成では通常、カップリング反応中のアミノ基の一時的な保護のために、Fmoc(9-フルオレニルメチルオキシカルボニル)化学を使用します。一般的なステップには以下が含まれます。
脱保護: ピペリジンなどの塩基を使用してFmoc基を除去します。
カップリング: HBTU(O-ベンゾトリアゾール-N、N、N'、N'-テトラメチルウロニウムヘキサフルオロリン酸)などのカップリング試薬を、DIPEA(N、N-ジイソプロピルエチルアミン)などの塩基の存在下で使用して、次のグリシン残基を添加します。
切断: トリフルオロ酢酸(TFA)、水、およびトリイソプロピルシラン(TIS)などのスカベンジャーの混合物を使用して、樹脂からペプチドを最終的に切断します。
工業生産方法: グリシン6は主に研究目的で合成されますが、工業規模の生産では、より大きなバッチサイズに合わせて最適化された同様のSPPS技術が採用されます。自動化と高スループットペプチドシンセサイザーは、効率と収率を高めるために使用できます。
化学反応の分析
反応の種類: グリシン6は、さまざまな化学反応を起こす可能性があり、以下が含まれます。
加水分解: 強い酸または塩基の存在下で個々のグリシン残基に分解されます。
酸化: 特定の条件下で、グリシン残基はグリオキシル酸とアンモニアに酸化される可能性があります。
置換: グリシン6のアミノ基は、求電子試薬との置換反応に関与できます。
一般的な試薬と条件:
加水分解: 通常、塩酸または水酸化ナトリウムを高温で使用して実行されます。
酸化: 過酸化水素または過マンガン酸カリウムなどの酸化剤を使用して達成できます。
置換: アシルクロリドまたはイソシアネートなどの求電子試薬を穏やかな条件で使用できます。
主な生成物:
加水分解: 遊離グリシン残基を生成します。
酸化: グリオキシル酸とアンモニアをもたらします。
置換: 使用される求電子試薬に応じて、さまざまな置換グリシン誘導体を形成します。
4. 科学研究への応用
グリシン6は、科学研究において幅広い用途があります。
生化学: タンパク質のフォールディング、安定性、相互作用を研究するためのモデルペプチドとして使用されます。
生物物理学: ペプチドのダイナミクスとコンフォメーションの変化を理解するための実験で使用されます。
医学: ドラッグデリバリーシステムにおける可能性のある役割と、治療用ペプチドのビルディングブロックとして調査されています。
科学的研究の応用
Gly6 has a wide range of applications in scientific research:
Biochemistry: Used as a model peptide to study protein folding, stability, and interactions.
Biophysics: Employed in experiments to understand peptide dynamics and conformational changes.
Medicine: Investigated for its potential role in drug delivery systems and as a building block for therapeutic peptides.
Industry: Utilized in the development of peptide-based materials and as a substrate in enzymatic studies.
作用機序
グリシン6の作用機序は、主にペプチドとしての構造的特性に関連しています。それは、水素結合、静電相互作用、疎水性効果を通じて、さまざまな生体分子と相互作用できます。酵素研究では、グリシン6は、グリシン残基間のペプチド結合を切断するプロテアーゼの基質として機能します。 切断生成物は、その後、さらなる生化学的経路に関与することができます .
類似の化合物:
グリシン2(ジグリシン): 2つのグリシン残基で構成されます。
グリシン3(トリグリシン): 3つのグリシン残基で構成されます。
グリシン4(テトラグリシン): 4つのグリシン残基で構成されます。
グリシン5(ペンタグリシン): 5つのグリシン残基で構成されます。
比較:
長さおよび複雑さ: グリシン6は、そのより短い対応物よりも長く複雑であり、その溶解性、安定性、および他の分子との相互作用に影響を与える可能性があります。
生物活性: グリシン6の長さが長くなると、生物学的標的とのより広範な相互作用が可能になり、研究および治療用途でのユーティリティが向上する可能性があります。
独自の特性: グリシン6の繰り返しグリシン配列は、ペプチドの挙動と相互作用を研究するための独自のモデルを提供し、より短いグリシンペプチドとは異なります.
類似化合物との比較
Gly2 (diglycine): Composed of two glycine residues.
Gly3 (triglycine): Composed of three glycine residues.
Gly4 (tetraglycine): Composed of four glycine residues.
Gly5 (pentaglycine): Composed of five glycine residues.
Comparison:
Length and Complexity: Gly6 is longer and more complex than its shorter counterparts, which can influence its solubility, stability, and interactions with other molecules.
Biological Activity: The increased length of Gly6 allows for more extensive interactions with biological targets, potentially enhancing its utility in research and therapeutic applications.
Unique Properties: Gly6’s repetitive glycine sequence provides a unique model for studying peptide behavior and interactions, making it distinct from shorter glycine peptides.
生物活性
Hexaglycine, a hexapeptide composed entirely of glycine residues, is of significant interest in biochemical research due to its unique properties and biological activities. This article explores the biological activity of this compound, focusing on its synthesis, structural characteristics, interactions, and potential applications in various fields.
This compound (Gly6) is a homopeptide consisting of six glycine amino acids linked by peptide bonds. Glycine is the simplest amino acid, and its repetitive structure in this compound leads to unique conformational properties that influence its biological activity. Research has indicated that this compound may play roles in various biological processes, including protein folding, cellular signaling, and as a model compound for studying peptide interactions.
2. Synthesis and Structural Properties
This compound can be synthesized through various methods, including chemical synthesis and enzymatic processes. The oligomerization of glycine under specific conditions has been demonstrated to yield this compound effectively. For instance, thermal cycling at high temperatures and alkaline pH has been shown to promote the formation of longer glycine chains, including this compound .
Table 1: Conditions for this compound Synthesis
Method | Conditions | Yield (%) |
---|---|---|
Thermal Cycling | 250°C, pH 9 | Variable |
Enzymatic Synthesis | Specific enzyme catalysis | High |
Chemical Synthesis | Coupling agents at room temperature | Moderate |
3.1 Interaction with Proteins
This compound exhibits significant interactions with proteins and other peptides. Its ability to form hydrogen bonds and hydrophobic interactions contributes to its role in stabilizing protein structures. Studies have shown that this compound can influence the phase separation of proteins in solution, which is critical for cellular processes such as signal transduction and metabolic regulation .
3.2 Cellular Uptake
Research indicates that this compound enhances the uptake of glycine into cells compared to free glycine. This property suggests that this compound may act as a carrier for glycine transport across cell membranes, potentially influencing metabolic pathways related to amino acid metabolism .
3.3 Anti-infective Properties
This compound has been investigated for its anti-infective properties against various pathogens. It has shown efficacy in inhibiting bacterial growth and may serve as a scaffold for developing new antibiotics or therapeutic agents targeting viral infections .
4.1 Study on Solubility and Aggregation
A case study examined the solubility and aggregation behavior of this compound in aqueous solutions. It was found that this compound tends to aggregate under certain conditions, which can affect its bioavailability and activity . The study highlighted the importance of environmental factors such as pH and ionic strength on the solubility of peptides.
4.2 Phase Separation in Cellular Contexts
Another study explored how this compound influences liquid-liquid phase separation (LLPS) in cellular environments. It was observed that under specific conditions, this compound could induce phase separation in proteins, leading to the formation of condensates that play critical roles in cellular organization .
5. Conclusion
This compound is a versatile peptide with notable biological activities that warrant further investigation. Its capacity to influence protein interactions, enhance cellular uptake of glycine, and exhibit potential anti-infective properties positions it as a valuable compound in biochemical research and therapeutic development.
特性
IUPAC Name |
2-[[2-[[2-[[2-[[2-[(2-aminoacetyl)amino]acetyl]amino]acetyl]amino]acetyl]amino]acetyl]amino]acetic acid | |
---|---|---|
Source | PubChem | |
URL | https://pubchem.ncbi.nlm.nih.gov | |
Description | Data deposited in or computed by PubChem | |
InChI |
InChI=1S/C12H20N6O7/c13-1-7(19)14-2-8(20)15-3-9(21)16-4-10(22)17-5-11(23)18-6-12(24)25/h1-6,13H2,(H,14,19)(H,15,20)(H,16,21)(H,17,22)(H,18,23)(H,24,25) | |
Source | PubChem | |
URL | https://pubchem.ncbi.nlm.nih.gov | |
Description | Data deposited in or computed by PubChem | |
InChI Key |
XJFPXLWGZWAWRQ-UHFFFAOYSA-N | |
Source | PubChem | |
URL | https://pubchem.ncbi.nlm.nih.gov | |
Description | Data deposited in or computed by PubChem | |
Canonical SMILES |
C(C(=O)NCC(=O)NCC(=O)NCC(=O)NCC(=O)NCC(=O)O)N | |
Source | PubChem | |
URL | https://pubchem.ncbi.nlm.nih.gov | |
Description | Data deposited in or computed by PubChem | |
Molecular Formula |
C12H20N6O7 | |
Source | PubChem | |
URL | https://pubchem.ncbi.nlm.nih.gov | |
Description | Data deposited in or computed by PubChem | |
DSSTOX Substance ID |
DTXSID90192125 | |
Record name | Hexaglycine | |
Source | EPA DSSTox | |
URL | https://comptox.epa.gov/dashboard/DTXSID90192125 | |
Description | DSSTox provides a high quality public chemistry resource for supporting improved predictive toxicology. | |
Molecular Weight |
360.32 g/mol | |
Source | PubChem | |
URL | https://pubchem.ncbi.nlm.nih.gov | |
Description | Data deposited in or computed by PubChem | |
CAS No. |
3887-13-6 | |
Record name | Hexaglycine | |
Source | ChemIDplus | |
URL | https://pubchem.ncbi.nlm.nih.gov/substance/?source=chemidplus&sourceid=0003887136 | |
Description | ChemIDplus is a free, web search system that provides access to the structure and nomenclature authority files used for the identification of chemical substances cited in National Library of Medicine (NLM) databases, including the TOXNET system. | |
Record name | 3887-13-6 | |
Source | DTP/NCI | |
URL | https://dtp.cancer.gov/dtpstandard/servlet/dwindex?searchtype=NSC&outputformat=html&searchlist=402061 | |
Description | The NCI Development Therapeutics Program (DTP) provides services and resources to the academic and private-sector research communities worldwide to facilitate the discovery and development of new cancer therapeutic agents. | |
Explanation | Unless otherwise indicated, all text within NCI products is free of copyright and may be reused without our permission. Credit the National Cancer Institute as the source. | |
Record name | Hexaglycine | |
Source | EPA DSSTox | |
URL | https://comptox.epa.gov/dashboard/DTXSID90192125 | |
Description | DSSTox provides a high quality public chemistry resource for supporting improved predictive toxicology. | |
Record name | HEXAGLYCINE | |
Source | FDA Global Substance Registration System (GSRS) | |
URL | https://gsrs.ncats.nih.gov/ginas/app/beta/substances/53RE43SN65 | |
Description | The FDA Global Substance Registration System (GSRS) enables the efficient and accurate exchange of information on what substances are in regulated products. Instead of relying on names, which vary across regulatory domains, countries, and regions, the GSRS knowledge base makes it possible for substances to be defined by standardized, scientific descriptions. | |
Explanation | Unless otherwise noted, the contents of the FDA website (www.fda.gov), both text and graphics, are not copyrighted. They are in the public domain and may be republished, reprinted and otherwise used freely by anyone without the need to obtain permission from FDA. Credit to the U.S. Food and Drug Administration as the source is appreciated but not required. | |
Retrosynthesis Analysis
AI-Powered Synthesis Planning: Our tool employs the Template_relevance Pistachio, Template_relevance Bkms_metabolic, Template_relevance Pistachio_ringbreaker, Template_relevance Reaxys, Template_relevance Reaxys_biocatalysis model, leveraging a vast database of chemical reactions to predict feasible synthetic routes.
One-Step Synthesis Focus: Specifically designed for one-step synthesis, it provides concise and direct routes for your target compounds, streamlining the synthesis process.
Accurate Predictions: Utilizing the extensive PISTACHIO, BKMS_METABOLIC, PISTACHIO_RINGBREAKER, REAXYS, REAXYS_BIOCATALYSIS database, our tool offers high-accuracy predictions, reflecting the latest in chemical research and data.
Strategy Settings
Precursor scoring | Relevance Heuristic |
---|---|
Min. plausibility | 0.01 |
Model | Template_relevance |
Template Set | Pistachio/Bkms_metabolic/Pistachio_ringbreaker/Reaxys/Reaxys_biocatalysis |
Top-N result to add to graph | 6 |
Feasible Synthetic Routes
Q1: What is the molecular formula and weight of Hexaglycine?
A1: this compound, with six glycine residues (C2H5NO2) linked together, possesses a molecular formula of C12H20N6O7 and a molecular weight of 360.31 g/mol.
Q2: Is there spectroscopic data available for this compound?
A2: Yes, studies utilizing techniques like circular dichroism (CD) spectroscopy have provided insights into the conformational behavior of this compound and its analogs. For instance, while exhibiting a random coil conformation in water, an analog of ACTH-(1-24)-tetracosapeptide containing this compound displayed alpha-helical characteristics in trifluoroethanol, highlighting the impact of solvent environment on its structure [].
Q3: How does the flexibility of this compound influence its end-to-end distance distribution?
A3: Research employing frequency-domain fluorescence spectroscopy has revealed that the flexibility of the peptide chain significantly impacts the end-to-end distance distribution in this compound. Specifically, the flexible nature of glycine results in a higher degree of conformational heterogeneity compared to more rigid peptides like Hexaproline. This difference in flexibility translates to a wider distribution of distances between the donor and acceptor molecules attached at the ends of the this compound chain [].
Q4: How does the presence of this compound in a fusion protein affect the protein's half-life?
A4: Studies have shown that incorporating this compound as a linker in a reiterated form of rabbit serum albumin (RSA) did not significantly alter the protein's half-life compared to a single unit of RSA []. This suggests that while albumin itself can extend the half-life of smaller proteins, simply reiterating it with a this compound linker might not confer additional benefits in terms of half-life extension.
Q5: Is the this compound peptide bond stable under strong alkaline conditions during peptide synthesis?
A5: Yes, a key advantage of using the bulky ferrocenylmethyl (Fem) group to protect glycine residues during peptide synthesis is the remarkable stability of the Gly-Gly bond in the presence of strong alkaline conditions, typically encountered during methyl ester hydrolysis steps []. This stability ensures high yields throughout the synthesis process.
Q6: Can this compound be used as a substrate to study the activity of lysostaphin-like bacteriolytic agents?
A6: Yes, this compound serves as a valuable tool for investigating the mode of action of bacteriolytic agents like zoocin A, produced by Streptococcus zooepidemicus. By observing zoocin A's ability to cleave this compound in a cell-free system, researchers have gathered evidence supporting its lysostaphin-like activity, suggesting that zoocin A targets and cleaves peptidoglycan cross-links in bacterial cell walls [].
Q7: How does the length of glycine oligopeptides affect their hydrolysis by intestinal enzymes?
A7: Studies on guinea pig intestinal mucosa have shown a correlation between the length of glycine oligopeptides and their susceptibility to enzymatic hydrolysis. While the mucosa exhibited hydrolytic activity for oligopeptides up to this compound, it did not show any activity against larger homopolypeptides []. This suggests a size limitation for the peptidase enzymes involved in the hydrolysis of glycine chains.
Q8: What is the role of this compound in studying the transport of glycine peptides in the human jejunum?
A8: Research using this compound has been crucial in determining the limits of intact absorption of glycine oligopeptides in the human jejunum. Results indicate that while di- and triglycine are readily absorbed, the uptake of glycine from this compound is comparable to that from free glycine. This suggests that longer glycine peptides are primarily hydrolyzed before absorption, highlighting the importance of peptide length for efficient nutrient uptake in the gut [].
Q9: How is this compound used in computational chemistry for studying peptide aggregation?
A9: this compound serves as a model peptide for investigating the aggregation behavior of polypeptide chains using density functional theory (DFT) calculations. By simulating the interactions of this compound strands in various conformations, researchers can gain insights into the energetic contributions of hydrogen bonding and structural distortions to the stability of different aggregated forms, such as pleated and rippled beta-sheets, collagen-like structures, and polyglycine helices [].
Q10: Can genetic algorithms be used to determine the low-energy conformations of this compound?
A10: Yes, genetic algorithms (GA) have been successfully employed to explore the conformational space of cyclic this compound and identify its low-energy conformations []. This approach involves utilizing GA parameters such as population size, number of generations, and interaction rates to effectively search for energetically favorable structures within a computationally feasible timeframe.
試験管内研究製品の免責事項と情報
BenchChemで提示されるすべての記事および製品情報は、情報提供を目的としています。BenchChemで購入可能な製品は、生体外研究のために特別に設計されています。生体外研究は、ラテン語の "in glass" に由来し、生物体の外で行われる実験を指します。これらの製品は医薬品または薬として分類されておらず、FDAから任何の医療状態、病気、または疾患の予防、治療、または治癒のために承認されていません。これらの製品を人間または動物に体内に導入する形態は、法律により厳格に禁止されています。これらのガイドラインに従うことは、研究と実験において法的および倫理的な基準の遵守を確実にするために重要です。