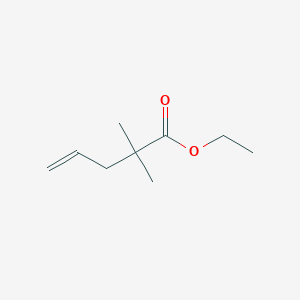
Ethyl 2,2-dimethylpent-4-enoate
概要
説明
Ethyl 2,2-dimethylpent-4-enoate is an organic compound with the molecular formula C9H16O2 and a molecular weight of 156.22 g/mol . It is an ester derived from 2,2-dimethylpent-4-enoic acid and ethanol. This compound is known for its applications in organic synthesis and as a building block in the production of various chemicals.
準備方法
Ethyl 2,2-dimethylpent-4-enoate can be synthesized through several methods. One common synthetic route involves the reaction of allyl bromide with ethyl isobutyrate . The reaction is typically carried out in the presence of a base such as sodium hydride or potassium carbonate, which facilitates the formation of the ester bond. The reaction conditions often include refluxing the reactants in an appropriate solvent like tetrahydrofuran (THF) or dimethylformamide (DMF) to achieve high yields.
Industrial production methods may involve similar synthetic routes but on a larger scale, with optimized reaction conditions to ensure cost-effectiveness and efficiency. The use of continuous flow reactors and advanced purification techniques can further enhance the production process.
化学反応の分析
Ethyl 2,2-dimethylpent-4-enoate undergoes various chemical reactions, including:
Oxidation: This compound can be oxidized to form corresponding carboxylic acids or ketones. Common oxidizing agents include potassium permanganate (KMnO4) and chromium trioxide (CrO3).
Reduction: Reduction reactions can convert the ester into alcohols. Reagents like lithium aluminium hydride (LiAlH4) or sodium borohydride (NaBH4) are commonly used for this purpose.
Substitution: Nucleophilic substitution reactions can occur at the ester group, leading to the formation of different esters or amides. Reagents such as ammonia or primary amines can be used under appropriate conditions.
Addition: The double bond in the compound allows for addition reactions, such as hydroboration-oxidation, which can convert the alkene into an alcohol.
The major products formed from these reactions depend on the specific reagents and conditions used. For example, oxidation with KMnO4 can yield carboxylic acids, while reduction with LiAlH4 produces alcohols.
科学的研究の応用
Ethyl 2,2-dimethylpent-4-enoate has several scientific research applications:
Chemistry: It is used as a building block in organic synthesis, enabling the creation of more complex molecules. Its reactivity makes it valuable in the development of new synthetic methodologies.
Biology: The compound can be used in the synthesis of biologically active molecules, including pharmaceuticals and agrochemicals. Its ester functionality is often found in prodrugs, which are inactive compounds that can be metabolized into active drugs in the body.
Medicine: Research into the compound’s potential therapeutic applications is ongoing. It may serve as a precursor for the synthesis of drugs targeting specific diseases.
Industry: this compound is used in the production of specialty chemicals, including flavors, fragrances, and polymers. Its unique structure allows for the creation of products with specific properties.
作用機序
The mechanism of action of ethyl 2,2-dimethylpent-4-enoate depends on its specific application. In organic synthesis, its reactivity is primarily due to the ester group and the double bond, which can undergo various chemical transformations. The molecular targets and pathways involved in these reactions are determined by the specific reagents and conditions used.
In biological systems, the compound’s ester group can be hydrolyzed by enzymes such as esterases, releasing the corresponding acid and alcohol. This hydrolysis is a key step in the activation of prodrugs, allowing the active drug to exert its therapeutic effects.
類似化合物との比較
Ethyl 2,2-dimethylpent-4-enoate can be compared with other similar compounds, such as:
Mthis compound: This compound has a similar structure but with a methyl ester group instead of an ethyl ester group. The difference in ester groups can affect the compound’s reactivity and physical properties.
Ethyl 2,2-dimethylpent-4-ynoate:
Ethyl 2-methyl-4-pentenoate: This compound has a similar structure but with a different substitution pattern on the carbon chain. The position of the methyl group can influence the compound’s reactivity and the types of reactions it undergoes.
This compound is unique due to its specific combination of functional groups and substitution pattern, which confer distinct reactivity and applications in various fields.
特性
IUPAC Name |
ethyl 2,2-dimethylpent-4-enoate | |
---|---|---|
Details | Computed by LexiChem 2.6.6 (PubChem release 2019.06.18) | |
Source | PubChem | |
URL | https://pubchem.ncbi.nlm.nih.gov | |
Description | Data deposited in or computed by PubChem | |
InChI |
InChI=1S/C9H16O2/c1-5-7-9(3,4)8(10)11-6-2/h5H,1,6-7H2,2-4H3 | |
Details | Computed by InChI 1.0.5 (PubChem release 2019.06.18) | |
Source | PubChem | |
URL | https://pubchem.ncbi.nlm.nih.gov | |
Description | Data deposited in or computed by PubChem | |
InChI Key |
HZVDMEDBEMVERD-UHFFFAOYSA-N | |
Details | Computed by InChI 1.0.5 (PubChem release 2019.06.18) | |
Source | PubChem | |
URL | https://pubchem.ncbi.nlm.nih.gov | |
Description | Data deposited in or computed by PubChem | |
Canonical SMILES |
CCOC(=O)C(C)(C)CC=C | |
Details | Computed by OEChem 2.1.5 (PubChem release 2019.06.18) | |
Source | PubChem | |
URL | https://pubchem.ncbi.nlm.nih.gov | |
Description | Data deposited in or computed by PubChem | |
Molecular Formula |
C9H16O2 | |
Details | Computed by PubChem 2.1 (PubChem release 2019.06.18) | |
Source | PubChem | |
URL | https://pubchem.ncbi.nlm.nih.gov | |
Description | Data deposited in or computed by PubChem | |
Molecular Weight |
156.22 g/mol | |
Details | Computed by PubChem 2.1 (PubChem release 2021.05.07) | |
Source | PubChem | |
URL | https://pubchem.ncbi.nlm.nih.gov | |
Description | Data deposited in or computed by PubChem | |
Retrosynthesis Analysis
AI-Powered Synthesis Planning: Our tool employs the Template_relevance Pistachio, Template_relevance Bkms_metabolic, Template_relevance Pistachio_ringbreaker, Template_relevance Reaxys, Template_relevance Reaxys_biocatalysis model, leveraging a vast database of chemical reactions to predict feasible synthetic routes.
One-Step Synthesis Focus: Specifically designed for one-step synthesis, it provides concise and direct routes for your target compounds, streamlining the synthesis process.
Accurate Predictions: Utilizing the extensive PISTACHIO, BKMS_METABOLIC, PISTACHIO_RINGBREAKER, REAXYS, REAXYS_BIOCATALYSIS database, our tool offers high-accuracy predictions, reflecting the latest in chemical research and data.
Strategy Settings
Precursor scoring | Relevance Heuristic |
---|---|
Min. plausibility | 0.01 |
Model | Template_relevance |
Template Set | Pistachio/Bkms_metabolic/Pistachio_ringbreaker/Reaxys/Reaxys_biocatalysis |
Top-N result to add to graph | 6 |
Feasible Synthetic Routes
試験管内研究製品の免責事項と情報
BenchChemで提示されるすべての記事および製品情報は、情報提供を目的としています。BenchChemで購入可能な製品は、生体外研究のために特別に設計されています。生体外研究は、ラテン語の "in glass" に由来し、生物体の外で行われる実験を指します。これらの製品は医薬品または薬として分類されておらず、FDAから任何の医療状態、病気、または疾患の予防、治療、または治癒のために承認されていません。これらの製品を人間または動物に体内に導入する形態は、法律により厳格に禁止されています。これらのガイドラインに従うことは、研究と実験において法的および倫理的な基準の遵守を確実にするために重要です。