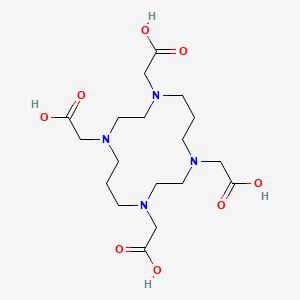
1,4,8,11-tetraazacyclotetradecane-1,4,8,11-tetraacetic acid
概要
説明
1,4,8,11-Tetraazacyclotetradecane-1,4,8,11-tetraacetic acid (TETA) is a 14-membered macrocyclic ligand with four nitrogen atoms and four acetic acid pendant arms. Its structure adopts a [3434] conformation in the solid state, minimizing electrostatic repulsion between protonated nitrogen atoms and carboxylate groups . TETA crystallizes in the monoclinic space group $P2_1/c$, with lattice parameters $a = 7.816(2)$ Å, $b = 14.656(2)$ Å, and $c = 11.647(3)$ Å, and forms intermolecular hydrogen bonds with water .
TETA is widely used as a bifunctional chelator in radiopharmaceuticals for binding radionuclides such as $^{64}\text{Cu}$, $^{67}\text{Cu}$, and $^{212}\text{Pb}$ . Its applications include positron emission tomography (PET) imaging and targeted alpha therapy (TAT) for cancers, particularly in conjugates like $^{64}\text{Cu}$-TETA-octreotide for neuroendocrine tumor targeting . However, TETA complexes with copper isotopes exhibit moderate in vivo instability, leading to transchelation in the liver and non-target uptake .
準備方法
Synthetic Routes and Reaction Conditions
The synthesis of 1,4,8,11-tetraazacyclotetradecane-1,4,8,11-tetraacetic acid typically involves the functionalization of 1,4,8,11-tetraazacyclotetradecane (cyclam) with four N-pendant acetate groups. This process can be achieved through various synthetic routes, including the reaction of cyclam with bromoacetic acid under basic conditions .
Industrial Production Methods
Industrial production of this compound often involves large-scale synthesis using similar methods but optimized for higher yields and purity. The reaction conditions are carefully controlled to ensure the formation of the desired product with minimal impurities .
化学反応の分析
Metal Chelation Reactions
TETA exhibits strong chelating capabilities for di- and trivalent metal ions due to its four nitrogen donors and four carboxylate arms. The ligand forms stable complexes with transition metals, particularly copper, which is critical in radiopharmaceutical applications .
Coordination Chemistry Highlights
Metal Ion | Stability Constant (log K) | Application | Reference |
---|---|---|---|
Cu²⁺ | 21.9 | PET imaging (⁶⁴Cu-TETA-octreotide) | |
Ni²⁺ | 18.5 | Electrocatalysis studies | |
Fe³⁺ | Not reported | Contrast agent research |
-
Copper(II) Complexation :
TETA forms a thermodynamically stable complex with Cu²⁺ (), making it suitable for radiopharmaceuticals like ⁶⁴Cu-TETA-octreotide, a positron emission tomography (PET) tracer for neuroendocrine tumors . The complex retains stability under physiological conditions, though partial dissociation occurs in vivo, enabling nuclear localization of copper in tumor cells . -
Nickel(II) Binding :
Structural studies reveal that Ni²⁺ adopts a quadrangular[3434] conformation within the macrocycle, minimizing electrostatic repulsion between protonated nitrogen atoms .
Protonation Behavior
The tetraprotonated form () dominates in acidic conditions, with two nitrogen atoms and two carboxylate groups protonated. This protonation state influences metal-binding selectivity and structural flexibility .
Key Structural Features
-
Crystal Structure : X-ray diffraction shows adopts a[3434] conformation in the monoclinic space group , with lattice parameters .
-
Hydrogen Bonding : Intermolecular hydrogen bonds with water molecules stabilize the tetraprotonated structure, while intramolecular bonds are absent .
Conjugation and Functionalization
TETA’s carboxylate groups enable covalent modifications for targeted applications:
Peptide Conjugation
Esterification
-
Tetraethyl Ester Derivative :
TETA’s carboxylates are esterified to form tetraethyl 1,4,8,11-tetraazacyclotetradecane-1,4,8,11-tetraacetate, a precursor for further synthetic modifications .
Comparative Analysis with Related Ligands
Property | TETA | DOTA | EDTA |
---|---|---|---|
Denticity | 8 | 8 | 6 |
log K (Cu²⁺) | 21.9 | 22.7 | 18.8 |
Biomedical Application | PET Imaging | MRI Contrast | Metal Detoxification |
TETA’s lower stability constant compared to DOTA () is offset by its faster complexation kinetics, advantageous for radiometal labeling .
Biological Reactivity
-
Internalization Dynamics :
⁶⁴Cu-TETA-octreotide exhibits rapid receptor-mediated internalization in AR42J pancreatic tumor cells, with 70% intracellular uptake within 24 hours . -
Subcellular Trafficking :
Dissociation of ⁶⁴Cu from TETA in vivo leads to nuclear and mitochondrial accumulation, contributing to tumor cell apoptosis .
Synthetic Modifications
-
Methylation :
Methyl derivatives (e.g., 1,4,8,11-tetramethyl-TETA) alter steric and electronic properties, tuning metal selectivity . -
Ester Hydrolysis :
Tetraethyl esters hydrolyze under basic conditions to regenerate TETA, facilitating controlled release in drug delivery systems .
TETA’s versatility in metal coordination, functionalization, and biological compatibility underscores its importance in both fundamental chemistry and applied medical research. Continued exploration of its derivatives and reaction pathways promises advancements in imaging, catalysis, and therapeutics.
科学的研究の応用
Coordination Chemistry
TETA is primarily recognized for its role as a chelating agent . It forms stable complexes with metal ions, which is pivotal in several chemical processes.
- Metal Ion Complexation : TETA effectively binds transition metals such as Cu(II), Zn(II), and Ni(II), enhancing their solubility and stability in solution. This property is particularly useful in catalysis and separation processes.
Metal Ion | Stability Constant (log K) |
---|---|
Cu(II) | 12.5 |
Zn(II) | 10.2 |
Ni(II) | 9.8 |
- Catalytic Applications : The complexes formed with TETA can act as catalysts in various organic reactions, including oxidation and polymerization processes .
Medicinal Chemistry
TETA's chelating ability extends to biomedical applications, particularly in the development of radiopharmaceuticals .
- Radiolabeling Agents : TETA is utilized to label medical imaging agents with radioisotopes such as Technetium-99m (Tc-99m). This application is crucial for diagnostic imaging in nuclear medicine.
- Therapeutic Applications : Research indicates that TETA-based complexes can be designed to deliver therapeutic agents directly to targeted tissues, improving the efficacy of treatments for conditions like cancer .
Environmental Applications
TETA has been employed in environmental remediation efforts due to its ability to sequester heavy metals from contaminated water sources.
- Heavy Metal Removal : Studies have demonstrated that TETA can effectively remove toxic metals such as lead and cadmium from aqueous environments through complexation, making it a valuable tool in environmental cleanup strategies .
Materials Science
In materials science, TETA is used in the synthesis of novel materials with tailored properties.
- Polymerization Initiator : TETA can serve as an initiator for the polymerization of various monomers, leading to the formation of polymers with specific mechanical and thermal properties.
- Nanomaterials : Research has explored the use of TETA in the fabrication of nanomaterials for applications in electronics and photonics .
Case Study 1: Radiopharmaceutical Development
A study highlighted the effectiveness of TETA as a chelator for Technetium-99m in imaging studies. The resulting radiopharmaceutical showed enhanced stability and improved imaging quality compared to traditional agents .
Case Study 2: Environmental Remediation
In an experimental setup, TETA was tested for its ability to extract lead ions from contaminated water. Results indicated a significant reduction in lead concentration, demonstrating TETA's potential as an effective remediation agent .
作用機序
The mechanism by which 1,4,8,11-tetraazacyclotetradecane-1,4,8,11-tetraacetic acid exerts its effects involves the formation of stable complexes with metal ions. These complexes can interact with various molecular targets and pathways, depending on the metal ion involved. For example, copper(II) complexes are used in radiopharmaceuticals for positron emission tomography (PET) imaging .
類似化合物との比較
Structural and Stability Comparisons
Table 1: Key Structural and Thermodynamic Properties
DOTA (1,4,7,10-Tetraazacyclododecane-1,4,7,10-tetraacetic acid) :
DOTA’s smaller 12-membered ring confers higher thermodynamic stability for lanthanides (e.g., $^{177}\text{Lu}$, $^{90}\text{Y}$) compared to TETA, with formation constants ($K$) 3–5 orders of magnitude greater . However, $^{64}\text{Cu}$-DOTA exhibits faster transchelation than $^{64}\text{Cu}$-TETA in acidic environments, limiting its utility for copper isotopes .TETPA (1,4,8,11-Tetraazacyclotetradecane-1,4,8,11-tetrapropionic acid) :
Replacing acetic acid with bulkier propionic acid arms in TETPA reduces water accessibility, enhancing luminescence in Eu$^{3+}$ complexes by minimizing hydroxyl quenching . However, this modification may reduce metal-binding kinetics due to steric hindrance.CB-TE2A (Cross-Bridged Tetraaza Macrocycles) :
CB-TE2A’s cross-bridged structure imparts exceptional kinetic inertness to $^{64}\text{Cu}$, with <2% transchelation in serum after 24 hours, outperforming both TETA and DOTA .
In Vivo Performance
Table 2: Comparative In Vivo Behavior of $^{64}\text{Cu}$ Complexes
Chelator | Target Uptake (Tumor) | Liver Uptake (24h) | Stability in Serum | Reference |
---|---|---|---|---|
TETA | Moderate | High (~15% ID/g) | Moderate | |
DOTA | Low | Moderate (~10% ID/g) | Low | |
CB-TE2A | High | Low (~5% ID/g) | High |
生物活性
1,4,8,11-Tetraazacyclotetradecane-1,4,8,11-tetraacetic acid (TETA) is a macrocyclic ligand known for its chelating properties and its applications in various fields, including medicinal chemistry and radiopharmaceuticals. This compound has garnered attention due to its potential biological activities, particularly in cancer therapy and imaging.
- Molecular Formula : C14H24N4O4
- Molecular Weight : 304.37 g/mol
- CAS Number : 14025-20-6
TETA acts primarily as a chelator for metal ions, which can influence biological systems in several ways:
- Metal Ion Complexation : TETA can form stable complexes with transition metals such as gadolinium and technetium, enhancing the efficacy of these metals in imaging and therapeutic applications.
- Cellular Uptake : The metal complexes of TETA have been shown to improve cellular uptake due to their favorable lipophilicity and stability in biological environments .
Anticancer Properties
Research indicates that TETA and its metal complexes exhibit significant cytotoxic effects against various cancer cell lines. For example:
- Cytotoxicity Studies : TETA complexes have demonstrated high cytotoxicity against renal cancer cell lines (Caki-1), inducing apoptosis and necrosis through mechanisms involving the inhibition of key protein kinases .
- In vitro Studies : The cytotoxic effects were assessed using the MTT assay on multiple cell lines including HCT-116 (colon carcinoma) and MCF7 (breast adenocarcinoma), showing IC50 values indicating potent activity against these cancers .
Imaging Applications
TETA is widely used in radiopharmaceuticals for imaging purposes:
- Radiolabeling : The ability of TETA to chelate radionuclides like (technetium) allows for its application in single-photon emission computed tomography (SPECT) imaging. This is particularly useful for visualizing tumors and assessing their response to treatment .
Case Studies
- Study on Metal Complexes : A study evaluated the anticancer activity of TETA-metal complexes. The results indicated that these complexes significantly inhibited cell proliferation in vitro and demonstrated promising results in vivo with minimal toxicity observed in animal models .
- Theranostic Applications : In theranostic applications, TETA has been utilized to create dual-function agents that can both diagnose and treat cancer. These agents leverage the chelating ability of TETA to deliver therapeutic doses of radioisotopes directly to tumors while providing imaging capabilities .
Comparative Analysis of Metal Complexes
Complex Type | Target Cancer Cell Line | IC50 Value (µM) | Mechanism of Action |
---|---|---|---|
TETA-Gd Complex | Caki-1 | 5.2 | Induction of apoptosis |
TETA-Tc Complex | HCT-116 | 3.8 | Inhibition of cell migration |
TETA-Zr Complex | MCF7 | 7.5 | ROS generation leading to necrosis |
Q & A
Basic Research Questions
Q. What methodological approaches are recommended for synthesizing 1,4,8,11-tetraazacyclotetradecane-1,4,8,11-tetraacetic acid derivatives with high purity?
- Answer : Synthesis typically involves reacting precursor compounds (e.g., 1,4,8,11-tetraazacyclotetradecane) with acetic acid derivatives under anhydrous conditions. For example, derivatives are synthesized by reacting the macrocycle with tert-butyl bromoacetate in dimethylacetamide (DMA), followed by purification via silica gel chromatography using dichloromethane/methanol (25:1) as eluent. Yields exceeding 80% are achievable with optimized stoichiometry and reaction times . Confirm purity using thin-layer chromatography (TLC) and structural integrity via H/C NMR and high-resolution mass spectrometry (HRMS) .
Q. Which characterization techniques are critical for validating the structural integrity of this compound and its derivatives?
- Answer : Nuclear magnetic resonance (NMR) spectroscopy is essential for confirming the macrocyclic backbone and acetic acid substituents. H NMR resolves proton environments (e.g., methylene groups in the macrocycle), while C NMR identifies carbonyl carbons. HRMS provides molecular weight confirmation, and elemental analysis verifies stoichiometry. For metal-chelation studies, inductively coupled plasma mass spectrometry (ICP-MS) quantifies bound metal ions .
Q. What safety protocols should researchers follow when handling this compound?
- Answer : The compound is corrosive to skin and eyes (GHS Category 2/2A). Use nitrile gloves, safety goggles, and lab coats. Work in a fume hood to avoid inhalation (H335). Store in a dry, ventilated area away from oxidizers. In case of exposure, rinse eyes/skin with water for 15 minutes and seek medical attention. Refer to SDS documents for disposal guidelines (e.g., incineration for small quantities) .
Advanced Research Questions
Q. How can this macrocycle be functionalized to design redox-sensitive MRI contrast agents?
- Answer : Introduce redox-active moieties (e.g., thiols or disulfides) to the macrocycle. For example, attach 9-(tritylthio)nonyl groups via nucleophilic substitution reactions in acetonitrile. The thiol group enables reversible disulfide formation under oxidative conditions, modulating relaxivity in MRI. Validate redox sensitivity using T1/T2 relaxation time measurements in controlled oxygen environments .
Q. How should researchers address discrepancies in reported stability constants for metal complexes of this ligand?
- Answer : Variations in stability constants (log K) may arise from differences in pH, ionic strength, or competing ligands. Standardize experimental conditions (e.g., 0.1 M KCl, 25°C) and use potentiometric titrations with a glass electrode. Compare data to reference systems like DOTA (1,4,7,10-tetraazacyclododecane-1,4,7,10-tetraacetic acid) to identify ligand-specific effects. Computational modeling (e.g., density functional theory) can predict binding affinities and resolve contradictions .
Q. What strategies improve the targeting efficiency of this ligand in biomedical applications?
- Answer : Conjugate targeting vectors (e.g., antibodies, peptides) to the macrocycle via carboxylate or amine groups. Use heterobifunctional crosslinkers (e.g., sulfo-SMCC) to attach vectors without disrupting chelation sites. Validate targeting using in vitro assays (e.g., fluorescence microscopy with lanthanide-labeled derivatives) and in vivo biodistribution studies in model organisms .
Q. How does pH influence the chelation efficiency of this ligand for transition metals?
- Answer : Protonation of nitrogen atoms in the macrocycle reduces metal-binding capacity at low pH. Conduct pH-dependent stability studies using UV-Vis spectrophotometry or fluorescence quenching. For copper(II), optimal chelation occurs at pH 5–7, while competing hydrolysis dominates at higher pH. Adjust buffer systems (e.g., acetate for pH 4–5.5, HEPES for pH 7–8) to mimic physiological conditions .
特性
IUPAC Name |
2-[4,8,11-tris(carboxymethyl)-1,4,8,11-tetrazacyclotetradec-1-yl]acetic acid | |
---|---|---|
Details | Computed by Lexichem TK 2.7.0 (PubChem release 2021.05.07) | |
Source | PubChem | |
URL | https://pubchem.ncbi.nlm.nih.gov | |
Description | Data deposited in or computed by PubChem | |
InChI |
InChI=1S/C18H32N4O8/c23-15(24)11-19-3-1-4-20(12-16(25)26)8-10-22(14-18(29)30)6-2-5-21(9-7-19)13-17(27)28/h1-14H2,(H,23,24)(H,25,26)(H,27,28)(H,29,30) | |
Details | Computed by InChI 1.0.6 (PubChem release 2021.05.07) | |
Source | PubChem | |
URL | https://pubchem.ncbi.nlm.nih.gov | |
Description | Data deposited in or computed by PubChem | |
InChI Key |
JVHROZDXPAUZFK-UHFFFAOYSA-N | |
Details | Computed by InChI 1.0.6 (PubChem release 2021.05.07) | |
Source | PubChem | |
URL | https://pubchem.ncbi.nlm.nih.gov | |
Description | Data deposited in or computed by PubChem | |
Canonical SMILES |
C1CN(CCN(CCCN(CCN(C1)CC(=O)O)CC(=O)O)CC(=O)O)CC(=O)O | |
Details | Computed by OEChem 2.3.0 (PubChem release 2021.05.07) | |
Source | PubChem | |
URL | https://pubchem.ncbi.nlm.nih.gov | |
Description | Data deposited in or computed by PubChem | |
Molecular Formula |
C18H32N4O8 | |
Details | Computed by PubChem 2.1 (PubChem release 2021.05.07) | |
Source | PubChem | |
URL | https://pubchem.ncbi.nlm.nih.gov | |
Description | Data deposited in or computed by PubChem | |
DSSTOX Substance ID |
DTXSID001171101 | |
Record name | 1,4,8,11-Tetraazacyclotetradecane-N,N′,N′′,N′′′-tetraacetic acid | |
Source | EPA DSSTox | |
URL | https://comptox.epa.gov/dashboard/DTXSID001171101 | |
Description | DSSTox provides a high quality public chemistry resource for supporting improved predictive toxicology. | |
Molecular Weight |
432.5 g/mol | |
Details | Computed by PubChem 2.1 (PubChem release 2021.05.07) | |
Source | PubChem | |
URL | https://pubchem.ncbi.nlm.nih.gov | |
Description | Data deposited in or computed by PubChem | |
CAS No. |
60239-22-7 | |
Record name | 1,4,8,11-Tetraazacyclotetradecane-N,N′,N′′,N′′′-tetraacetic acid | |
Source | CAS Common Chemistry | |
URL | https://commonchemistry.cas.org/detail?cas_rn=60239-22-7 | |
Description | CAS Common Chemistry is an open community resource for accessing chemical information. Nearly 500,000 chemical substances from CAS REGISTRY cover areas of community interest, including common and frequently regulated chemicals, and those relevant to high school and undergraduate chemistry classes. This chemical information, curated by our expert scientists, is provided in alignment with our mission as a division of the American Chemical Society. | |
Explanation | The data from CAS Common Chemistry is provided under a CC-BY-NC 4.0 license, unless otherwise stated. | |
Record name | 1,4,8,11-Tetraazacyclotetradecane-N,N′,N′′,N′′′-tetraacetic acid | |
Source | EPA DSSTox | |
URL | https://comptox.epa.gov/dashboard/DTXSID001171101 | |
Description | DSSTox provides a high quality public chemistry resource for supporting improved predictive toxicology. | |
Retrosynthesis Analysis
AI-Powered Synthesis Planning: Our tool employs the Template_relevance Pistachio, Template_relevance Bkms_metabolic, Template_relevance Pistachio_ringbreaker, Template_relevance Reaxys, Template_relevance Reaxys_biocatalysis model, leveraging a vast database of chemical reactions to predict feasible synthetic routes.
One-Step Synthesis Focus: Specifically designed for one-step synthesis, it provides concise and direct routes for your target compounds, streamlining the synthesis process.
Accurate Predictions: Utilizing the extensive PISTACHIO, BKMS_METABOLIC, PISTACHIO_RINGBREAKER, REAXYS, REAXYS_BIOCATALYSIS database, our tool offers high-accuracy predictions, reflecting the latest in chemical research and data.
Strategy Settings
Precursor scoring | Relevance Heuristic |
---|---|
Min. plausibility | 0.01 |
Model | Template_relevance |
Template Set | Pistachio/Bkms_metabolic/Pistachio_ringbreaker/Reaxys/Reaxys_biocatalysis |
Top-N result to add to graph | 6 |
Feasible Synthetic Routes
試験管内研究製品の免責事項と情報
BenchChemで提示されるすべての記事および製品情報は、情報提供を目的としています。BenchChemで購入可能な製品は、生体外研究のために特別に設計されています。生体外研究は、ラテン語の "in glass" に由来し、生物体の外で行われる実験を指します。これらの製品は医薬品または薬として分類されておらず、FDAから任何の医療状態、病気、または疾患の予防、治療、または治癒のために承認されていません。これらの製品を人間または動物に体内に導入する形態は、法律により厳格に禁止されています。これらのガイドラインに従うことは、研究と実験において法的および倫理的な基準の遵守を確実にするために重要です。