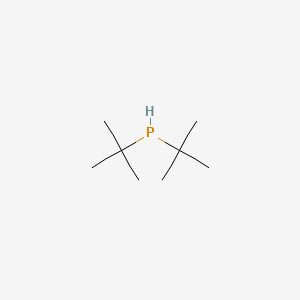
Di-tert-butylphosphine
概要
説明
Di-tert-butylphosphine is a bulky secondary phosphine ligand . It is also known as Bis (tert-butyl)phosphine . It has a linear formula of (CH3)3CPHC(CH3)3 and a molecular weight of 146.21 .
Synthesis Analysis
Di-tert-butylphosphine can be used in the synthesis of a variety of ligands, such as Q-Phos . It is used for C-N, C-O, and C-C bond-forming cross-coupling reactions .Molecular Structure Analysis
The molecular structure of Di-tert-butylphosphine is represented by the SMILES string CC©©PC©©C . It is a mixed carbon- and phosphorus-based tetrahedral molecule .Chemical Reactions Analysis
Di-tert-butylphosphine is suitable for various types of reactions. It is used as a ligand in Buchwald-Hartwig Cross Coupling Reaction, Heck Reaction, and Suzuki-Miyaura Coupling .Physical And Chemical Properties Analysis
Di-tert-butylphosphine is a liquid at room temperature . It has a density of 0.79 g/mL at 25 °C .科学的研究の応用
- Di-tert-butylphosphine serves as a ligand in metal complexes, enhancing their catalytic activity. It is commonly used in palladium-catalyzed reactions, such as Suzuki-Miyaura cross-couplings of arylboronic acids with aryl bromides and chlorides .
- The bulky tBu₂PH group stabilizes metal complexes, influencing their reactivity and selectivity. For example, it contributes to the effectiveness of JohnPhos ligands in cross-coupling reactions .
- Di-tert-butylphosphine participates in C-C bond-forming reactions. It is employed in the synthesis of various ligands, including Q-Phos, which facilitates cross-coupling reactions (C-N, C-O, and C-C bonds) .
- tBu₂PH is a key component in the decarboxylative cross-coupling of dialkoxybenzoic acids with diaryl disulfides or diaryl diselenides .
- Di-tert-butylphosphine enables the hydrophenoxylation of unactivated internal alkynes, a valuable synthetic transformation .
- Researchers have used tBu₂PH in the pharmaceutical synthesis of novel imidazo[1,2-a]pyridines, which exhibit potent activity against the herpes virus .
Homogeneous Catalysis
Cross-Coupling Reactions
Decarboxylative Cross-Coupling
Hydrophenoxylation of Alkynes
Imidazo[1,2-a]pyridine Synthesis
Chiral Asymmetric Hydrogenations
作用機序
Target of Action
Di-tert-butylphosphine primarily targets central metal atoms in various chemical reactions . It plays a key role in stabilizing and activating these atoms, which are crucial for the success of the reactions .
Mode of Action
Di-tert-butylphosphine interacts with its targets through its flexible electronic and steric properties . This interaction enables the compound to stabilize and activate the central metal atom, thereby facilitating various types of reactions, including transition metal-catalyzed C-O, C-N, and C-C bond-forming reactions .
Biochemical Pathways
Di-tert-butylphosphine is involved in a wide range of tervalent phosphorus ligands in transition metal catalysis and organocatalysis . These pathways inspire the design of new phosphines of various structures and the tuning of their properties .
Pharmacokinetics
It’s known that the compound isair sensitive , which may influence its absorption, distribution, metabolism, and excretion (ADME) properties.
Result of Action
The action of Di-tert-butylphosphine results in the formation of new bonds in the target molecules . This can lead to the synthesis of a variety of ligands, such as Q-Phos, for C-N, C-O, and C-C bond-forming cross-coupling reactions .
Action Environment
The action of Di-tert-butylphosphine is influenced by environmental factors . For instance, it’s known to be air sensitive , which means its action, efficacy, and stability can be affected by exposure to air. Moreover, it’s used in many organometallic synthetic and catalytic applications, suggesting that the reaction environment can significantly influence its action .
Safety and Hazards
将来の方向性
特性
IUPAC Name |
ditert-butylphosphane | |
---|---|---|
Details | Computed by Lexichem TK 2.7.0 (PubChem release 2021.05.07) | |
Source | PubChem | |
URL | https://pubchem.ncbi.nlm.nih.gov | |
Description | Data deposited in or computed by PubChem | |
InChI |
InChI=1S/C8H19P/c1-7(2,3)9-8(4,5)6/h9H,1-6H3 | |
Details | Computed by InChI 1.0.6 (PubChem release 2021.05.07) | |
Source | PubChem | |
URL | https://pubchem.ncbi.nlm.nih.gov | |
Description | Data deposited in or computed by PubChem | |
InChI Key |
CRHWEIDCXNDTMO-UHFFFAOYSA-N | |
Details | Computed by InChI 1.0.6 (PubChem release 2021.05.07) | |
Source | PubChem | |
URL | https://pubchem.ncbi.nlm.nih.gov | |
Description | Data deposited in or computed by PubChem | |
Canonical SMILES |
CC(C)(C)PC(C)(C)C | |
Details | Computed by OEChem 2.3.0 (PubChem release 2021.05.07) | |
Source | PubChem | |
URL | https://pubchem.ncbi.nlm.nih.gov | |
Description | Data deposited in or computed by PubChem | |
Molecular Formula |
C8H19P | |
Details | Computed by PubChem 2.1 (PubChem release 2021.05.07) | |
Source | PubChem | |
URL | https://pubchem.ncbi.nlm.nih.gov | |
Description | Data deposited in or computed by PubChem | |
DSSTOX Substance ID |
DTXSID40231452 | |
Record name | Phosphine, bis(1,1-dimethylethyl)- | |
Source | EPA DSSTox | |
URL | https://comptox.epa.gov/dashboard/DTXSID40231452 | |
Description | DSSTox provides a high quality public chemistry resource for supporting improved predictive toxicology. | |
Molecular Weight |
146.21 g/mol | |
Details | Computed by PubChem 2.1 (PubChem release 2021.05.07) | |
Source | PubChem | |
URL | https://pubchem.ncbi.nlm.nih.gov | |
Description | Data deposited in or computed by PubChem | |
Product Name |
Di-tert-butylphosphine | |
CAS RN |
819-19-2 | |
Record name | Phosphine, bis(1,1-dimethylethyl)- | |
Source | ChemIDplus | |
URL | https://pubchem.ncbi.nlm.nih.gov/substance/?source=chemidplus&sourceid=0000819192 | |
Description | ChemIDplus is a free, web search system that provides access to the structure and nomenclature authority files used for the identification of chemical substances cited in National Library of Medicine (NLM) databases, including the TOXNET system. | |
Record name | Phosphine, bis(1,1-dimethylethyl)- | |
Source | EPA DSSTox | |
URL | https://comptox.epa.gov/dashboard/DTXSID40231452 | |
Description | DSSTox provides a high quality public chemistry resource for supporting improved predictive toxicology. | |
Record name | Di-t-butylphosphine | |
Source | European Chemicals Agency (ECHA) | |
URL | https://echa.europa.eu/information-on-chemicals | |
Description | The European Chemicals Agency (ECHA) is an agency of the European Union which is the driving force among regulatory authorities in implementing the EU's groundbreaking chemicals legislation for the benefit of human health and the environment as well as for innovation and competitiveness. | |
Explanation | Use of the information, documents and data from the ECHA website is subject to the terms and conditions of this Legal Notice, and subject to other binding limitations provided for under applicable law, the information, documents and data made available on the ECHA website may be reproduced, distributed and/or used, totally or in part, for non-commercial purposes provided that ECHA is acknowledged as the source: "Source: European Chemicals Agency, http://echa.europa.eu/". Such acknowledgement must be included in each copy of the material. ECHA permits and encourages organisations and individuals to create links to the ECHA website under the following cumulative conditions: Links can only be made to webpages that provide a link to the Legal Notice page. | |
Synthesis routes and methods I
Procedure details
Synthesis routes and methods II
Procedure details
Retrosynthesis Analysis
AI-Powered Synthesis Planning: Our tool employs the Template_relevance Pistachio, Template_relevance Bkms_metabolic, Template_relevance Pistachio_ringbreaker, Template_relevance Reaxys, Template_relevance Reaxys_biocatalysis model, leveraging a vast database of chemical reactions to predict feasible synthetic routes.
One-Step Synthesis Focus: Specifically designed for one-step synthesis, it provides concise and direct routes for your target compounds, streamlining the synthesis process.
Accurate Predictions: Utilizing the extensive PISTACHIO, BKMS_METABOLIC, PISTACHIO_RINGBREAKER, REAXYS, REAXYS_BIOCATALYSIS database, our tool offers high-accuracy predictions, reflecting the latest in chemical research and data.
Strategy Settings
Precursor scoring | Relevance Heuristic |
---|---|
Min. plausibility | 0.01 |
Model | Template_relevance |
Template Set | Pistachio/Bkms_metabolic/Pistachio_ringbreaker/Reaxys/Reaxys_biocatalysis |
Top-N result to add to graph | 6 |
Feasible Synthetic Routes
Q & A
Q1: What is the molecular formula, weight, and key spectroscopic data for di-tert-butylphosphine?
A1: Di-tert-butylphosphine has the molecular formula C₈H₁₉P and a molecular weight of 146.23 g/mol. Key spectroscopic data include:
- ³¹P NMR: A singlet is typically observed in the range of δ 15-65 ppm, depending on the solvent and substituents on the phosphorus atom. For example, 1-adamantyl di-tert-butylphosphine exhibits a signal at δ 63.0 ppm in C₆D₆. []
Q2: What are the structural features that make di-tert-butylphosphine unique as a ligand?
A2: Di-tert-butylphosphine is characterized by its significant steric bulk due to the two tert-butyl groups attached to the phosphorus atom. This bulkiness leads to a strong preference for low coordination numbers at the metal center and can promote the formation of coordinatively unsaturated and highly reactive metal complexes.
Q3: What types of reactions are catalyzed by metal complexes containing di-tert-butylphosphine as a ligand?
A3: Di-tert-butylphosphine-containing metal complexes have demonstrated high activity and selectivity in a broad range of reactions, including:
- Palladium-catalyzed cross-coupling reactions: Suzuki-Miyaura coupling, [, , , , , , ] Buchwald-Hartwig amination, [, , , ] α-arylation of carbonyl compounds, [, , , , ] and Sonogashira coupling. []
- Gold-catalyzed cycloisomerization reactions: Formation of various heterocycles and carbocycles from enynes and diynes. [, , , , , ]
Q4: How does the steric bulk of di-tert-butylphosphine influence the activity and selectivity of palladium catalysts in α-arylation reactions?
A5: The significant steric bulk of di-tert-butylphosphine promotes the formation of monoligated palladium species, which are more reactive in oxidative addition steps compared to bisligated complexes. This feature allows for milder reaction conditions and higher selectivity for monoarylation products, even at elevated temperatures. [, ]
Q5: Can you elaborate on the use of di-tert-butylphosphine-based ligands in gold catalysis and their impact on reaction selectivity?
A6: Gold(I) complexes with di-tert-butylphosphine ligands, such as JohnPhos ((1,1'-biphenyl-2-yl)-di-tert-butylphosphine), have proven valuable in controlling the chemo- and regioselectivity of cycloisomerization reactions. The steric and electronic properties of the ligand can dictate the reaction pathway, leading to distinct product outcomes. For instance, depending on the ligand and reaction conditions, 1,6-diyne esters can be selectively converted to 1H-cyclopenta[b]naphthalenes, cis-cyclopenten-2-yl δ-diketones, or bicyclo[3.2.0]hepta-1,5-dienes. []
Q6: Have computational studies been conducted on di-tert-butylphosphine-containing systems?
A7: Yes, computational studies, including Density Functional Theory (DFT) calculations, have been employed to investigate the mechanisms of reactions catalyzed by di-tert-butylphosphine complexes. These calculations provide insights into the energetics and structures of intermediates and transition states, elucidating the factors governing reactivity and selectivity. [, ]
Q7: How do modifications to the di-tert-butylphosphine structure affect the catalytic activity of its metal complexes?
A8: Introducing substituents on the aryl groups of di-tert-butylphosphine-based ligands, like JohnPhos, can fine-tune the steric and electronic properties of the catalyst. For instance, employing a more sterically demanding ligand like Me4tBuXPhos (di-tert-butyl(2',4',6'-triisopropyl-3,4,5,6-tetramethyl-[1,1'-biphenyl]-2-yl)phosphine) can alter the reaction pathway in the gold(I)-catalyzed cycloisomerization of 1,11-dien-3,9-diyne benzoates, favoring the formation of bridged hexenone derivatives over bridged heptenones. []
Q8: Are there any strategies to improve the stability of di-tert-butylphosphine?
A8: While di-tert-butylphosphine itself is air-sensitive, its storage and handling can be facilitated by using solutions in inert solvents or storing it under an inert atmosphere.
Q9: What analytical techniques are commonly used to characterize di-tert-butylphosphine and its complexes?
A9: Characterization of di-tert-butylphosphine and its metal complexes typically involves a combination of techniques, including:
- Infrared (IR) Spectroscopy: Useful for identifying functional groups and studying metal-ligand interactions. [, ]
- X-ray Diffraction: Provides detailed information about the solid-state structure of crystalline complexes. [, , , , , ]
- Gas Chromatography-Infrared Spectroscopy/Mass Spectrometry (GC-FTIR/MS): Can be employed to identify and quantify di-tert-butylphosphine and its degradation products. []
Q10: Are there any alternative ligands to di-tert-butylphosphine for similar catalytic applications?
A12: A wide range of phosphine ligands with varying steric and electronic properties have been developed for similar catalytic applications. These include trialkylphosphines (e.g., tri-tert-butylphosphine), triarylphosphines (e.g., triphenylphosphine), and various bidentate phosphines (e.g., BINAP). [, ] The choice of ligand depends on the specific reaction and desired selectivity.
試験管内研究製品の免責事項と情報
BenchChemで提示されるすべての記事および製品情報は、情報提供を目的としています。BenchChemで購入可能な製品は、生体外研究のために特別に設計されています。生体外研究は、ラテン語の "in glass" に由来し、生物体の外で行われる実験を指します。これらの製品は医薬品または薬として分類されておらず、FDAから任何の医療状態、病気、または疾患の予防、治療、または治癒のために承認されていません。これらの製品を人間または動物に体内に導入する形態は、法律により厳格に禁止されています。これらのガイドラインに従うことは、研究と実験において法的および倫理的な基準の遵守を確実にするために重要です。