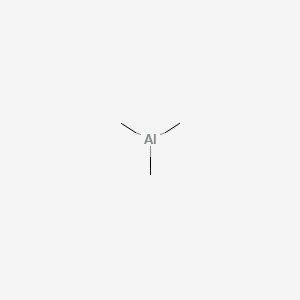
Trimethylaluminum
説明
Trimethylaluminum (TMA), with the molecular formula $ \text{C}3\text{H}9\text{Al} $, is a highly reactive organoaluminum compound. It is widely used in metalorganic chemical vapor deposition (MOCVD) for growing III-nitride semiconductors such as AlInGaN, AlN, and GaN . TMA serves as a critical aluminum precursor due to its volatility and efficient decomposition at moderate temperatures (~850°C) . Industrially, TMA is also employed in catalysis, polymer synthesis, and thin-film fabrication via atomic layer deposition (ALD) .
Key properties of TMA include:
- Pyrophoric nature: Reacts violently with air and moisture, requiring inert handling conditions .
- Lewis acidity: Forms stable adducts with Lewis bases like ammonia or ethers, enabling controlled reactivity in synthetic processes .
- Thermal decomposition: Produces aluminum-containing films (e.g., AlN, Al$2$O$3$) when reacted with oxygen or nitrogen sources .
準備方法
Trimethylaluminum is prepared via a two-step process. The first step involves the reaction of aluminum with methyl chloride in the presence of sodium, producing dimethylaluminum chloride. In the second step, dimethylaluminum chloride reacts with additional sodium to form this compound and sodium chloride .
化学反応の分析
Reaction with Ozone in Atomic Layer Deposition (ALD)
TMA reacts with ozone (O₃) during ALD processes to form aluminum oxide (Al₂O₃) surfaces. Density functional theory (DFT) calculations reveal a multi-step mechanism involving intermediates such as methoxy (−OCH₃), formate (−OCHO), bicarbonate (−CO₃H), and hydroxyl (−OH) species :
Reaction Step | Key Intermediate(s) | Activation Energy (kcal/mol) | Byproducts |
---|---|---|---|
Initial TMA + O₃ reaction | Al(CH₃)₂(OCH₃)(O₂) | 5.3 | CH₄, O₂ |
OCH₃ + O₂ interaction | Al(CH₃)₂(OCH₂)(O₂H) | 12.1 | CH₄, H₂O |
Formate formation | Al(CH₃)(OCHO)(OH) | 8.7 | CO, H₂O |
Bicarbonate generation | Al(CO₃H) | 10.5 | CO₂, H₂O |
These intermediates align with experimental observations of ALD byproducts (CH₄, CO₂, H₂O) . The reaction is highly exothermic, with TMA readily oxidizing even at low temperatures (35 K) .
Hydrolysis and Reactions with Protic Substances
TMA reacts violently with water, alcohols, acids, and amines due to its strong Lewis acidity and electrophilic Al center:
Hydrolysis
This reaction releases methane (CH₄) and forms aluminum oxide . Controlled hydrolysis yields methylaluminoxane (MAO), a co-catalyst in olefin polymerization .
Alcoholysis and Aminolysis
-
With ethanol :
-
With dimethylamine :
These reactions produce stable alkoxide or amide complexes .
Atomic Layer Etching (ALE) of Al₂O₃
TMA pairs with hydrogen fluoride (HF) in thermal ALE processes to etch Al₂O₃ via self-limiting reactions :
-
HF exposure : Fluorinates the Al₂O₃ surface to form AlF₃.
-
TMA exposure : Ligand-exchange reactions remove AlF₃ as volatile Al(CH₃)₃F₃.
Etching rates range from 0.5–1.2 Å/cycle at 250–325°C, demonstrating precise control over material removal .
Reaction with Atomic Nitrogen for Aluminum Nitride (AlN) Deposition
TMA reacts with atomic nitrogen (N) downstream of a N₂ plasma to deposit AlN thin films :
科学的研究の応用
Semiconductor Fabrication
TMA in Atomic Layer Deposition (ALD)
TMA is widely used in atomic layer deposition (ALD) processes to create thin films of aluminum oxide (Al2O3). This method is crucial for producing high-k dielectrics in semiconductor devices. The ALD process using TMA allows for precise control over film thickness and uniformity, making it ideal for applications in microelectronics.
- Case Study: ALD of Al2O3 using TMA
TMA in Metal-Organic Chemical Vapor Deposition (MOCVD)
In MOCVD, TMA serves as a precursor for aluminum-containing compound semiconductors such as AlN and AlGaN. These materials are essential for optoelectronic devices, including LEDs and laser diodes.
- Data Table: TMA Applications in Semiconductor Materials
Application | Material Type | Process Type | Key Benefits |
---|---|---|---|
ALD | Al2O3 | ALD | High uniformity, precise thickness |
MOCVD | AlN, AlGaN | MOCVD | High-quality epitaxial growth |
Epitaxy | AlInGaN | MOCVD | Enhanced UV luminescence |
Photovoltaic Applications
TMA is also utilized in the fabrication of photovoltaic devices. It aids in depositing low-k dielectric layers that enhance surface passivation of silicon substrates, improving device efficiency.
- Case Study: TMA in Perovskite Solar Cells
Chemical Synthesis
Reactivity with Organic Compounds
TMA is employed in various chemical reactions, including alkylation and ketonization processes. Its reactivity allows it to serve as a valuable reagent in organic synthesis.
- Case Study: Ketonization Reactions
Material Science
TMA-Doped Polymers
In material science, TMA has been investigated for its role in enhancing the properties of biodegradable polymers. Doping polymers with TMA can improve their optical and mechanical characteristics.
- Data Table: Effects of TMA on Polymer Properties
Polymer Type | Modification Method | Resulting Properties |
---|---|---|
Biodegradable | TMA Doping | Enhanced luminescence and mechanical strength |
Photoluminescent | Composite Formation | Improved optical properties |
作用機序
The mechanism of action of trimethylaluminum involves its high reactivity due to the presence of aluminum-carbon bonds. In chemical vapor deposition, this compound reacts with surface hydroxyl groups to form aluminum oxide and methane. This process involves ligand exchange reactions and the formation of intermediate species such as methoxy and formate groups .
類似化合物との比較
Comparison with Similar Organometallic Compounds
Trimethylaluminum vs. Trimethylgallium (TMGa) and Trimethylindium (TMIn)
TMA, TMGa, and TMIn are pivotal precursors in MOCVD for III-nitride growth. Their distinct roles and effects are compared below:
Parameter | This compound (TMA) | Trimethylgallium (TMGa) | Trimethylindium (TMIn) |
---|---|---|---|
Primary Role | Al source for AlN/AlGaN layers | Ga source for GaN/InGaN layers | In source for InGaN layers |
Thermal Stability | Decomposes at ~850°C | Decomposes at ~550–700°C | Decomposes at ~450–600°C |
Effect on Bandgap | Increases bandgap (AlN: ~6.2 eV) | Modulates bandgap (GaN: ~3.4 eV) | Reduces bandgap (InN: ~0.7 eV) |
Lattice Impact | High lattice mismatch with InN (~13%) | Moderate mismatch with InN (~10%) | Low mismatch with GaN (~2%) |
Phase Separation | Promotes InN segregation at high Al content | Minimal phase separation in Ga-rich alloys | Induces In-rich clusters in InGaN |
Key Findings :
- AlInGaN Growth : Increasing TMA flow rates (1.15–1.90 µmol/min) raise Al content (15–21.6%) but reduce In incorporation (4.2–3.4%) due to competing Al–N and In–N bond formation . Phase separation into InN domains occurs at higher TMA flows, evidenced by XRD peaks at 31.1° (InN) and Raman modes at 458 cm$^{-1}$ (InN A1(TO)) .
- Optical Properties : Al-rich AlInGaN (17.5% Al) exhibits enhanced UV emission (3.59–3.69 eV) due to carrier localization from In segregation, despite higher defect densities .
- Crystal Quality : TMA-driven Al incorporation broadens XRD FWHM (195–252 arcsec), indicating increased compositional disorder .
Reactivity Comparison with Other Organoaluminum Compounds
TMA’s reactivity differs from dimethylaluminum chloride (DMAC) and methylaluminoxane (MAO):
Key Insights :
- Surface Reactions : TMA adsorbs on γ-alumina as dimethylaluminum ($ \text{CH}3)2\text{Al} $), releasing methane upon annealing. DMAC shows similar pathways but faster kinetics .
- Catalytic Activity : MAO’s rigid cage structure enables efficient activation of metallocenes, unlike TMA, which requires co-catalysts for polymerization .
生物活性
Trimethylaluminum (TMA), a highly reactive organoaluminum compound, has garnered attention in various fields due to its unique chemical properties. Its biological activity, particularly in synthetic biology and materials science, has been explored in recent studies. This article reviews the biological implications of TMA, focusing on its applications in peptide synthesis, surface modification, and potential biocompatibility.
TMA is characterized by its ability to form stable complexes with various organic molecules. The compound acts as a Lewis acid, facilitating reactions with nucleophiles such as amino acids. Its reactivity is critical in synthesizing complex biomolecules and modifying surfaces for enhanced biocompatibility.
1. Peptide Synthesis
TMA has been successfully utilized in the synthesis of peptides through a one-pot reaction mechanism. This method involves the formation of five-membered cyclic intermediates that enhance the efficiency of peptide bond formation between unprotected amino acids. Notably, the reaction can accommodate bulky side chains, yielding high amounts of tripeptides without expensive coupling reagents .
Table 1: Summary of Peptide Synthesis Using TMA
Amino Acids Used | Reaction Conditions | Yield (%) |
---|---|---|
N-, C-terminal unprotected amino acids | One-pot synthesis with TMA | High |
Bulky side chain amino acids | Efficient coupling with TMA | High |
2. Surface Modification
TMA has been employed in atomic layer deposition (ALD) to modify the surfaces of various materials, including cellulose fibers. Research indicates that TMA exposure transforms hydrophilic surfaces to hydrophobic ones, enhancing mechanical properties such as tensile strength and elongation at break . This transformation is significant for applications in biomedical devices and tissue engineering.
Table 2: Effects of TMA on Surface Properties
Parameter | Before TMA Treatment | After TMA Treatment |
---|---|---|
Hydrophilicity | High | Low |
Tensile Strength (MPa) | X | Y |
Elongation at Break (%) | A | B |
Case Study 1: Peptide Bond Formation
In a study published in 2023, researchers demonstrated that TMA could effectively facilitate peptide bond formation between various amino acids. The study highlighted the advantages of using TMA over traditional coupling agents, showcasing its cost-effectiveness and efficiency in synthesizing complex peptides .
Case Study 2: Surface Hydrophilicity Modification
Another investigation focused on the transformation of cotton fiber surfaces via ALD using TMA. The study revealed that multiple cycles of TMA exposure significantly altered the mechanical properties of the fibers while reducing their surface energy, thus enhancing their applicability in hydrophobic coatings for biomedical applications .
Safety and Toxicity Considerations
Despite its beneficial applications, TMA poses safety risks due to its high reactivity and potential toxicity. Proper handling protocols are essential when working with this compound to mitigate risks associated with exposure.
Q & A
Basic Research Questions
Q. What are the key physicochemical properties of TMA critical for experimental design in organometallic chemistry?
TMA is highly pyrophoric, moisture-sensitive, and exists as a dimer in non-polar solvents but dissociates in coordinating solvents. Key properties include its Lewis acidity, vapor pressure (~10.4 mmHg at 20°C), and reactivity with protic substrates. Researchers must prioritize inert atmosphere techniques (e.g., Schlenk lines, gloveboxes) and solvent selection (e.g., toluene vs. ethers) to control dimerization and reactivity .
Q. How can researchers safely handle and store TMA in laboratory settings?
Use sealed stainless-steel cylinders under inert gas (argon/nitrogen), conduct manipulations in gloveboxes, and employ double-walled containment for liquid transfers. Personal protective equipment (PPE) must include flame-resistant lab coats, face shields, and neoprene gloves. Emergency protocols for spills (e.g., sand/vermiculite suppression) should align with SDS guidelines .
Q. What spectroscopic methods are most effective for characterizing TMA and its reaction intermediates?
- NMR : Use low-temperature ¹³C NMR to study monomer-dimer equilibria; chemical shifts at δ 8–10 ppm (Al–CH₃) and coupling constants (¹J(²⁷Al,¹³C) ≈ 100 Hz) reveal coordination changes .
- FTIR : Monitor Al–C stretching vibrations (500–600 cm⁻¹) and ligand-exchange reactions via peak shifts.
- Mass spectrometry : Cryogenic matrix-assisted techniques prevent thermal decomposition during analysis .
Q. Where can researchers access reliable thermodynamic data for TMA?
The NIST Chemistry WebBook provides validated thermochemical data (e.g., enthalpy of formation: ΔfH° = −238 kJ/mol) and Antoine equation parameters for vapor pressure modeling. Cross-reference with experimental measurements using calorimetry or gas-phase equilibria studies .
Advanced Research Questions
Q. How can experimental and computational methods resolve contradictions in TMA reaction mechanisms?
Conflicting reports on TMA’s reactivity (e.g., ALD growth rates, polymer infiltration kinetics) often arise from unaccounted variables like trace moisture or solvent coordination. Strategies include:
- ALD : Compare in situ QCM (quartz crystal microbalance) data with DFT simulations to validate surface reaction pathways (e.g., ligand exchange vs. disproportionation) .
- Polymer infiltration : Pair temperature-dependent FTIR with ab initio MD simulations to distinguish diffusion-limited vs. reaction-limited regimes in PMMA/TMA systems .
Q. What methodologies optimize TMA-based ALD processes for alumina thin films?
- Pulse parameters : Optimize TMA exposure time (0.1–1 s) and purge duration (5–20 s) using in situ ellipsometry to minimize precursor waste and ensure monolayer saturation .
- Co-reactants : Compare H₂O vs. O₃ for oxygen sources; O₃ yields higher purity Al₂O₃ but risks carbon contamination from incomplete ligand removal.
- Substrate pretreatment : Plasma activation (e.g., O₂ plasma) enhances nucleation density on hydrophobic surfaces .
Q. How do solvent effects influence TMA’s reactivity in organometallic synthesis?
Solvent Lewis basicity (e.g., Et₂O > toluene) shifts monomer-dimer equilibria and alters reaction kinetics. For example:
- In benzene, TMA quantitatively complexes with benzophenone, enabling rapid ketone alkylation.
- In Et₂O, solvent competition reduces complexation, requiring longer reaction times or elevated temperatures. Kinetic studies using UV-Vis spectroscopy (λ = 300–400 nm for charge-transfer bands) quantify these effects .
Q. What strategies mitigate challenges in quantifying TMA’s role in Ziegler-Natta catalysis?
- Poisoning studies : Introduce selective inhibitors (e.g., CO) to deactivate TMA co-catalysts and isolate their contribution to polymer chain initiation.
- Kinetic isotope effects : Compare H₂O vs. D₂O in hydrolysis reactions to probe rate-determining steps in catalyst activation .
Q. Data Contradiction Analysis
Q. How should researchers address discrepancies in reported TMA infiltration rates into polymers?
Discrepancies between PMMA and PLA infiltration studies stem from differences in polymer crystallinity and TMA’s diffusivity. Resolve by:
- Controlled annealing : Adjust polymer crystallinity (DSC analysis) and correlate with infiltration depth (XPS/SIMS profiling).
- Temperature gradients : Perform infiltration at 50–80°C (below polymer Tg) to isolate diffusion from chemical reaction effects .
Q. Why do computational models sometimes fail to predict TMA’s ALD growth rates accurately?
Over-simplified surface reaction mechanisms (e.g., ignoring steric effects or byproduct readsorption) cause deviations. Improve models by:
特性
IUPAC Name |
trimethylalumane | |
---|---|---|
Details | Computed by Lexichem TK 2.7.0 (PubChem release 2021.05.07) | |
Source | PubChem | |
URL | https://pubchem.ncbi.nlm.nih.gov | |
Description | Data deposited in or computed by PubChem | |
InChI |
InChI=1S/3CH3.Al/h3*1H3; | |
Details | Computed by InChI 1.0.6 (PubChem release 2021.05.07) | |
Source | PubChem | |
URL | https://pubchem.ncbi.nlm.nih.gov | |
Description | Data deposited in or computed by PubChem | |
InChI Key |
JLTRXTDYQLMHGR-UHFFFAOYSA-N | |
Details | Computed by InChI 1.0.6 (PubChem release 2021.05.07) | |
Source | PubChem | |
URL | https://pubchem.ncbi.nlm.nih.gov | |
Description | Data deposited in or computed by PubChem | |
Canonical SMILES |
C[Al](C)C | |
Details | Computed by OEChem 2.3.0 (PubChem release 2021.05.07) | |
Source | PubChem | |
URL | https://pubchem.ncbi.nlm.nih.gov | |
Description | Data deposited in or computed by PubChem | |
Molecular Formula |
C3H9Al | |
Details | Computed by PubChem 2.1 (PubChem release 2021.05.07) | |
Source | PubChem | |
URL | https://pubchem.ncbi.nlm.nih.gov | |
Description | Data deposited in or computed by PubChem | |
DSSTOX Substance ID |
DTXSID9058787 | |
Record name | Aluminum, trimethyl- | |
Source | EPA DSSTox | |
URL | https://comptox.epa.gov/dashboard/DTXSID9058787 | |
Description | DSSTox provides a high quality public chemistry resource for supporting improved predictive toxicology. | |
Molecular Weight |
72.09 g/mol | |
Details | Computed by PubChem 2.1 (PubChem release 2021.05.07) | |
Source | PubChem | |
URL | https://pubchem.ncbi.nlm.nih.gov | |
Description | Data deposited in or computed by PubChem | |
Physical Description |
Liquid, Clear colorless liquid; mp = 15 deg C; [Merck Index] | |
Record name | Aluminum, trimethyl- | |
Source | EPA Chemicals under the TSCA | |
URL | https://www.epa.gov/chemicals-under-tsca | |
Description | EPA Chemicals under the Toxic Substances Control Act (TSCA) collection contains information on chemicals and their regulations under TSCA, including non-confidential content from the TSCA Chemical Substance Inventory and Chemical Data Reporting. | |
Record name | Trimethylaluminum | |
Source | Haz-Map, Information on Hazardous Chemicals and Occupational Diseases | |
URL | https://haz-map.com/Agents/18353 | |
Description | Haz-Map® is an occupational health database designed for health and safety professionals and for consumers seeking information about the adverse effects of workplace exposures to chemical and biological agents. | |
Explanation | Copyright (c) 2022 Haz-Map(R). All rights reserved. Unless otherwise indicated, all materials from Haz-Map are copyrighted by Haz-Map(R). No part of these materials, either text or image may be used for any purpose other than for personal use. Therefore, reproduction, modification, storage in a retrieval system or retransmission, in any form or by any means, electronic, mechanical or otherwise, for reasons other than personal use, is strictly prohibited without prior written permission. | |
Vapor Pressure |
12.4 [mmHg] | |
Record name | Trimethylaluminum | |
Source | Haz-Map, Information on Hazardous Chemicals and Occupational Diseases | |
URL | https://haz-map.com/Agents/18353 | |
Description | Haz-Map® is an occupational health database designed for health and safety professionals and for consumers seeking information about the adverse effects of workplace exposures to chemical and biological agents. | |
Explanation | Copyright (c) 2022 Haz-Map(R). All rights reserved. Unless otherwise indicated, all materials from Haz-Map are copyrighted by Haz-Map(R). No part of these materials, either text or image may be used for any purpose other than for personal use. Therefore, reproduction, modification, storage in a retrieval system or retransmission, in any form or by any means, electronic, mechanical or otherwise, for reasons other than personal use, is strictly prohibited without prior written permission. | |
CAS No. |
75-24-1 | |
Record name | Trimethylaluminum | |
Source | CAS Common Chemistry | |
URL | https://commonchemistry.cas.org/detail?cas_rn=75-24-1 | |
Description | CAS Common Chemistry is an open community resource for accessing chemical information. Nearly 500,000 chemical substances from CAS REGISTRY cover areas of community interest, including common and frequently regulated chemicals, and those relevant to high school and undergraduate chemistry classes. This chemical information, curated by our expert scientists, is provided in alignment with our mission as a division of the American Chemical Society. | |
Explanation | The data from CAS Common Chemistry is provided under a CC-BY-NC 4.0 license, unless otherwise stated. | |
Record name | Trimethylaluminum | |
Source | ChemIDplus | |
URL | https://pubchem.ncbi.nlm.nih.gov/substance/?source=chemidplus&sourceid=0000075241 | |
Description | ChemIDplus is a free, web search system that provides access to the structure and nomenclature authority files used for the identification of chemical substances cited in National Library of Medicine (NLM) databases, including the TOXNET system. | |
Record name | Aluminum, trimethyl- | |
Source | EPA Chemicals under the TSCA | |
URL | https://www.epa.gov/chemicals-under-tsca | |
Description | EPA Chemicals under the Toxic Substances Control Act (TSCA) collection contains information on chemicals and their regulations under TSCA, including non-confidential content from the TSCA Chemical Substance Inventory and Chemical Data Reporting. | |
Record name | Aluminum, trimethyl- | |
Source | EPA DSSTox | |
URL | https://comptox.epa.gov/dashboard/DTXSID9058787 | |
Description | DSSTox provides a high quality public chemistry resource for supporting improved predictive toxicology. | |
Record name | Trimethylaluminium | |
Source | European Chemicals Agency (ECHA) | |
URL | https://echa.europa.eu/substance-information/-/substanceinfo/100.000.776 | |
Description | The European Chemicals Agency (ECHA) is an agency of the European Union which is the driving force among regulatory authorities in implementing the EU's groundbreaking chemicals legislation for the benefit of human health and the environment as well as for innovation and competitiveness. | |
Explanation | Use of the information, documents and data from the ECHA website is subject to the terms and conditions of this Legal Notice, and subject to other binding limitations provided for under applicable law, the information, documents and data made available on the ECHA website may be reproduced, distributed and/or used, totally or in part, for non-commercial purposes provided that ECHA is acknowledged as the source: "Source: European Chemicals Agency, http://echa.europa.eu/". Such acknowledgement must be included in each copy of the material. ECHA permits and encourages organisations and individuals to create links to the ECHA website under the following cumulative conditions: Links can only be made to webpages that provide a link to the Legal Notice page. | |
Record name | TRIMETHYLALUMINUM | |
Source | FDA Global Substance Registration System (GSRS) | |
URL | https://gsrs.ncats.nih.gov/ginas/app/beta/substances/AV210LG46J | |
Description | The FDA Global Substance Registration System (GSRS) enables the efficient and accurate exchange of information on what substances are in regulated products. Instead of relying on names, which vary across regulatory domains, countries, and regions, the GSRS knowledge base makes it possible for substances to be defined by standardized, scientific descriptions. | |
Explanation | Unless otherwise noted, the contents of the FDA website (www.fda.gov), both text and graphics, are not copyrighted. They are in the public domain and may be republished, reprinted and otherwise used freely by anyone without the need to obtain permission from FDA. Credit to the U.S. Food and Drug Administration as the source is appreciated but not required. | |
試験管内研究製品の免責事項と情報
BenchChemで提示されるすべての記事および製品情報は、情報提供を目的としています。BenchChemで購入可能な製品は、生体外研究のために特別に設計されています。生体外研究は、ラテン語の "in glass" に由来し、生物体の外で行われる実験を指します。これらの製品は医薬品または薬として分類されておらず、FDAから任何の医療状態、病気、または疾患の予防、治療、または治癒のために承認されていません。これらの製品を人間または動物に体内に導入する形態は、法律により厳格に禁止されています。これらのガイドラインに従うことは、研究と実験において法的および倫理的な基準の遵守を確実にするために重要です。