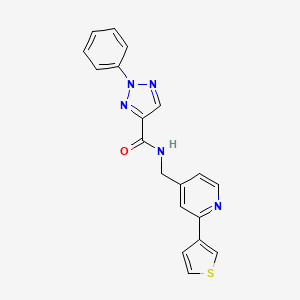
2-phenyl-N-((2-(thiophen-3-yl)pyridin-4-yl)methyl)-2H-1,2,3-triazole-4-carboxamide
- 専門家チームからの見積もりを受け取るには、QUICK INQUIRYをクリックしてください。
- 品質商品を競争力のある価格で提供し、研究に集中できます。
説明
2-phenyl-N-((2-(thiophen-3-yl)pyridin-4-yl)methyl)-2H-1,2,3-triazole-4-carboxamide is a useful research compound. Its molecular formula is C19H15N5OS and its molecular weight is 361.42. The purity is usually 95%.
BenchChem offers high-quality this compound suitable for many research applications. Different packaging options are available to accommodate customers' requirements. Please inquire for more information about this compound including the price, delivery time, and more detailed information at info@benchchem.com.
科学的研究の応用
Synthesis and Structural Assessment
The synthesis and structural assessment of related triazole compounds have been a focus of research, aiming to explore their potential applications. For instance, a study by Castiñeiras et al. (2018) discusses the synthesis of triazole derivatives and their complexation with metals such as mercury, highlighting the potential for creating novel materials with specific structural properties (Castiñeiras, García-Santos, & Saa, 2018). This approach can lead to the development of new materials with applications in catalysis, molecular recognition, and as components in electronic devices.
Heterocyclic Synthesis
Research into heterocyclic synthesis, like the work by Mohareb et al. (2004), demonstrates the versatility of triazole compounds in the creation of various heterocyclic structures (Mohareb, Sherif, Gaber, Ghabrial, & Aziz, 2004). These structures are crucial for pharmaceutical applications, offering potential as scaffolds for drug development due to their diverse biological activities.
Antimicrobial Activities
Another significant area of application is in the evaluation of antimicrobial activities. Bayrak et al. (2009) synthesized new 1,2,4-triazoles and investigated their antimicrobial properties (Bayrak, Demirbaş, Karaoglu, & Demirbas, 2009). The study revealed that these compounds exhibited good to moderate antimicrobial activity, indicating their potential use in developing new antimicrobial agents.
Anticancer and Anti-Inflammatory Applications
Research by Rahmouni et al. (2016) into novel pyrazolopyrimidines derivatives, which share structural similarities with the triazole compound , highlights the potential for anticancer and anti-inflammatory applications (Rahmouni, Souiei, Belkacem, Romdhane, & Bouajila, 2016). These findings suggest that triazole derivatives could be explored further for their efficacy in treating various diseases, including cancer and inflammation-related conditions.
Material Science and Electronic Devices
Additionally, the research conducted by Liu, Wang, & Yao (2018) on the molecular evolution of host materials for phosphorescent organic light-emitting diodes (PhOLEDs) demonstrates the potential application of triazole derivatives in electronic devices (Liu, Wang, & Yao, 2018). This study underscores the importance of such compounds in developing advanced materials for electronic applications, such as lighting and display technologies.
特性
IUPAC Name |
2-phenyl-N-[(2-thiophen-3-ylpyridin-4-yl)methyl]triazole-4-carboxamide |
Source
|
---|---|---|
Details | Computed by Lexichem TK 2.7.0 (PubChem release 2021.05.07) | |
Source | PubChem | |
URL | https://pubchem.ncbi.nlm.nih.gov | |
Description | Data deposited in or computed by PubChem | |
InChI |
InChI=1S/C19H15N5OS/c25-19(18-12-22-24(23-18)16-4-2-1-3-5-16)21-11-14-6-8-20-17(10-14)15-7-9-26-13-15/h1-10,12-13H,11H2,(H,21,25) |
Source
|
Details | Computed by InChI 1.0.6 (PubChem release 2021.05.07) | |
Source | PubChem | |
URL | https://pubchem.ncbi.nlm.nih.gov | |
Description | Data deposited in or computed by PubChem | |
InChI Key |
AZQORJKHSABYIW-UHFFFAOYSA-N |
Source
|
Details | Computed by InChI 1.0.6 (PubChem release 2021.05.07) | |
Source | PubChem | |
URL | https://pubchem.ncbi.nlm.nih.gov | |
Description | Data deposited in or computed by PubChem | |
Canonical SMILES |
C1=CC=C(C=C1)N2N=CC(=N2)C(=O)NCC3=CC(=NC=C3)C4=CSC=C4 |
Source
|
Details | Computed by OEChem 2.3.0 (PubChem release 2021.05.07) | |
Source | PubChem | |
URL | https://pubchem.ncbi.nlm.nih.gov | |
Description | Data deposited in or computed by PubChem | |
Molecular Formula |
C19H15N5OS |
Source
|
Details | Computed by PubChem 2.1 (PubChem release 2021.05.07) | |
Source | PubChem | |
URL | https://pubchem.ncbi.nlm.nih.gov | |
Description | Data deposited in or computed by PubChem | |
Molecular Weight |
361.4 g/mol |
Source
|
Details | Computed by PubChem 2.1 (PubChem release 2021.05.07) | |
Source | PubChem | |
URL | https://pubchem.ncbi.nlm.nih.gov | |
Description | Data deposited in or computed by PubChem | |
試験管内研究製品の免責事項と情報
BenchChemで提示されるすべての記事および製品情報は、情報提供を目的としています。BenchChemで購入可能な製品は、生体外研究のために特別に設計されています。生体外研究は、ラテン語の "in glass" に由来し、生物体の外で行われる実験を指します。これらの製品は医薬品または薬として分類されておらず、FDAから任何の医療状態、病気、または疾患の予防、治療、または治癒のために承認されていません。これらの製品を人間または動物に体内に導入する形態は、法律により厳格に禁止されています。これらのガイドラインに従うことは、研究と実験において法的および倫理的な基準の遵守を確実にするために重要です。