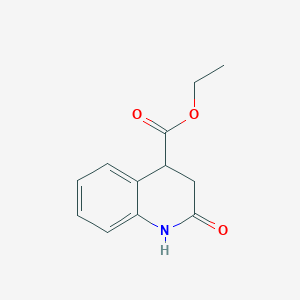
Ethyl 2-oxo-1,2,3,4-tetrahydroquinoline-4-carboxylate
- 専門家チームからの見積もりを受け取るには、QUICK INQUIRYをクリックしてください。
- 品質商品を競争力のある価格で提供し、研究に集中できます。
説明
Ethyl 2-oxo-1,2,3,4-tetrahydroquinoline-4-carboxylate is a heterocyclic compound that belongs to the quinoline family This compound is characterized by its unique structure, which includes a quinoline core with an ethyl ester group at the 4-position and a keto group at the 2-position
作用機序
Target of Action
Tetrahydroquinoline derivatives are known to have a wide range of applications and are key structural motifs in pharmaceutical agents .
Mode of Action
Ethyl 2-oxo-1,2,3,4-tetrahydroquinoline-4-carboxylate is synthesized through a three-component cascade reaction involving 2-alkenyl aniline, aldehydes, and ethyl cyanoacetate . The reaction proceeds through the Knoevenagel condensation of ethyl cyanoacetate with aldehydes followed by the aza-Michael–Michael addition with 2-alkenyl anilines to prepare the tetrahydroquinoline scaffolds .
Pharmacokinetics
The compound’s molecular weight is 21924 , which may influence its bioavailability and pharmacokinetic properties.
準備方法
Synthetic Routes and Reaction Conditions
Ethyl 2-oxo-1,2,3,4-tetrahydroquinoline-4-carboxylate can be synthesized through several methods. One common approach involves the cyclization of ortho-amino benzylidene malonates in the presence of Lewis acids such as boron trifluoride etherate (BF3·Et2O). This reaction proceeds through the formation of a stable iminium intermediate containing a difluoroboryl bridge in the dicarbonyl fragment of the molecule .
Industrial Production Methods
Industrial production methods for this compound typically involve large-scale synthesis using similar cyclization reactions. The use of efficient catalysts and optimized reaction conditions ensures high yields and purity of the final product. The process is designed to be cost-effective and scalable for commercial applications.
化学反応の分析
Types of Reactions
Ethyl 2-oxo-1,2,3,4-tetrahydroquinoline-4-carboxylate undergoes various chemical reactions, including:
Oxidation: The compound can be oxidized to form quinoline derivatives.
Reduction: Reduction reactions can convert the keto group to a hydroxyl group.
Substitution: The ester group can be substituted with other functional groups through nucleophilic substitution reactions.
Common Reagents and Conditions
Common reagents used in these reactions include oxidizing agents like potassium permanganate (KMnO4) for oxidation, reducing agents like sodium borohydride (NaBH4) for reduction, and nucleophiles like amines for substitution reactions. The reactions are typically carried out under controlled conditions to ensure selectivity and yield.
Major Products Formed
The major products formed from these reactions include various quinoline derivatives, hydroxylated compounds, and substituted esters. These products have diverse applications in different fields.
科学的研究の応用
Ethyl 2-oxo-1,2,3,4-tetrahydroquinoline-4-carboxylate has numerous scientific research applications:
Chemistry: It is used as a building block for the synthesis of more complex heterocyclic compounds.
Biology: The compound is studied for its potential biological activities, including antimicrobial and anticancer properties.
Medicine: Research is ongoing to explore its potential as a therapeutic agent for various diseases.
Industry: It is used in the production of pharmaceuticals, agrochemicals, and other fine chemicals.
類似化合物との比較
Ethyl 2-oxo-1,2,3,4-tetrahydroquinoline-4-carboxylate can be compared with other similar compounds, such as:
Indole derivatives: These compounds also have a heterocyclic structure and are known for their biological activities.
2-oxo-1,2,3,4-tetrahydropyrimidines: These compounds share a similar core structure and have applications in medicinal chemistry.
The uniqueness of this compound lies in its specific substitution pattern and the resulting chemical and biological properties.
特性
IUPAC Name |
ethyl 2-oxo-3,4-dihydro-1H-quinoline-4-carboxylate |
Source
|
---|---|---|
Details | Computed by LexiChem 2.6.6 (PubChem release 2019.06.18) | |
Source | PubChem | |
URL | https://pubchem.ncbi.nlm.nih.gov | |
Description | Data deposited in or computed by PubChem | |
InChI |
InChI=1S/C12H13NO3/c1-2-16-12(15)9-7-11(14)13-10-6-4-3-5-8(9)10/h3-6,9H,2,7H2,1H3,(H,13,14) |
Source
|
Details | Computed by InChI 1.0.5 (PubChem release 2019.06.18) | |
Source | PubChem | |
URL | https://pubchem.ncbi.nlm.nih.gov | |
Description | Data deposited in or computed by PubChem | |
InChI Key |
WSBUDAJUVYHBLM-UHFFFAOYSA-N |
Source
|
Details | Computed by InChI 1.0.5 (PubChem release 2019.06.18) | |
Source | PubChem | |
URL | https://pubchem.ncbi.nlm.nih.gov | |
Description | Data deposited in or computed by PubChem | |
Canonical SMILES |
CCOC(=O)C1CC(=O)NC2=CC=CC=C12 |
Source
|
Details | Computed by OEChem 2.1.5 (PubChem release 2019.06.18) | |
Source | PubChem | |
URL | https://pubchem.ncbi.nlm.nih.gov | |
Description | Data deposited in or computed by PubChem | |
Molecular Formula |
C12H13NO3 |
Source
|
Details | Computed by PubChem 2.1 (PubChem release 2019.06.18) | |
Source | PubChem | |
URL | https://pubchem.ncbi.nlm.nih.gov | |
Description | Data deposited in or computed by PubChem | |
Molecular Weight |
219.24 g/mol |
Source
|
Details | Computed by PubChem 2.1 (PubChem release 2021.05.07) | |
Source | PubChem | |
URL | https://pubchem.ncbi.nlm.nih.gov | |
Description | Data deposited in or computed by PubChem | |
試験管内研究製品の免責事項と情報
BenchChemで提示されるすべての記事および製品情報は、情報提供を目的としています。BenchChemで購入可能な製品は、生体外研究のために特別に設計されています。生体外研究は、ラテン語の "in glass" に由来し、生物体の外で行われる実験を指します。これらの製品は医薬品または薬として分類されておらず、FDAから任何の医療状態、病気、または疾患の予防、治療、または治癒のために承認されていません。これらの製品を人間または動物に体内に導入する形態は、法律により厳格に禁止されています。これらのガイドラインに従うことは、研究と実験において法的および倫理的な基準の遵守を確実にするために重要です。