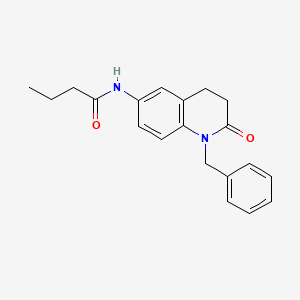
N-(1-benzyl-2-oxo-1,2,3,4-tetrahydroquinolin-6-yl)butanamide
- 専門家チームからの見積もりを受け取るには、QUICK INQUIRYをクリックしてください。
- 品質商品を競争力のある価格で提供し、研究に集中できます。
説明
N-(1-benzyl-2-oxo-1,2,3,4-tetrahydroquinolin-6-yl)butanamide is a synthetic organic compound that belongs to the class of quinoline derivatives This compound is characterized by its unique structure, which includes a quinoline core, a benzyl group, and a butanamide moiety
作用機序
Target of Action
It is known that indole derivatives, which share a similar structure with this compound, bind with high affinity to multiple receptors . This suggests that N-(1-benzyl-2-oxo-1,2,3,4-tetrahydroquinolin-6-yl)butanamide may also interact with various biological targets.
Mode of Action
Indole derivatives are known to interact with their targets in a way that leads to various biological activities, including antiviral, anti-inflammatory, anticancer, anti-hiv, antioxidant, antimicrobial, antitubercular, antidiabetic, antimalarial, and anticholinesterase activities . It is plausible that this compound may exhibit similar interactions and effects.
Biochemical Pathways
Given the broad spectrum of biological activities exhibited by similar indole derivatives , it is likely that this compound may affect multiple pathways and exert downstream effects on various biological processes.
Result of Action
Based on the known activities of similar indole derivatives , it can be inferred that this compound may have a wide range of effects at the molecular and cellular levels.
Action Environment
Environmental factors can significantly influence the action, efficacy, and stability of this compound . These factors can include the pH of the environment, the presence of other molecules or compounds, temperature, and more.
生化学分析
Biochemical Properties
N-(1-benzyl-2-oxo-1,2,3,4-tetrahydroquinolin-6-yl)butanamide plays a significant role in various biochemical reactions. It interacts with several enzymes, proteins, and other biomolecules. For instance, it has been shown to inhibit acetylcholine esterase (AChE), an enzyme responsible for breaking down the neurotransmitter acetylcholine . This inhibition can enhance cholinergic signaling, which is beneficial in treating conditions like Alzheimer’s disease. Additionally, this compound interacts with other proteins involved in cellular signaling pathways, potentially modulating their activity and influencing cellular responses.
Cellular Effects
This compound exerts various effects on different cell types and cellular processes. It has been observed to influence cell signaling pathways, gene expression, and cellular metabolism. For example, in neuronal cells, this compound can enhance cholinergic signaling by inhibiting acetylcholine esterase, leading to improved cognitive function . In cancer cells, this compound has shown cytotoxic effects, potentially through the induction of apoptosis and inhibition of cell proliferation . These cellular effects highlight the compound’s potential as a therapeutic agent for neurodegenerative diseases and cancer.
Molecular Mechanism
The molecular mechanism of this compound involves its interactions with various biomolecules. The compound binds to the active site of acetylcholine esterase, inhibiting its activity and preventing the breakdown of acetylcholine . This binding interaction enhances cholinergic signaling, which can improve cognitive function in neurodegenerative diseases. Additionally, this compound may interact with other enzymes and proteins involved in cellular signaling pathways, modulating their activity and influencing cellular responses. These interactions at the molecular level contribute to the compound’s therapeutic potential.
Temporal Effects in Laboratory Settings
In laboratory settings, the effects of this compound can change over time. The compound’s stability and degradation are important factors to consider. Studies have shown that this compound remains stable under certain conditions, but it may degrade over time, leading to a decrease in its efficacy . Long-term effects on cellular function have also been observed, with prolonged exposure to the compound resulting in sustained inhibition of acetylcholine esterase activity and enhanced cholinergic signaling. These temporal effects highlight the importance of considering the stability and duration of treatment when using this compound in research and therapeutic applications.
Dosage Effects in Animal Models
The effects of this compound vary with different dosages in animal models. At lower doses, the compound has been shown to enhance cognitive function by inhibiting acetylcholine esterase and improving cholinergic signaling . At higher doses, toxic or adverse effects may be observed, including potential neurotoxicity and hepatotoxicity. These dosage effects highlight the importance of determining the optimal dose for therapeutic applications while minimizing potential side effects.
Metabolic Pathways
This compound is involved in various metabolic pathways. It interacts with enzymes and cofactors that play a role in its metabolism and clearance from the body. For instance, the compound may be metabolized by cytochrome P450 enzymes, leading to the formation of metabolites that are subsequently excreted . These metabolic pathways can influence the compound’s pharmacokinetics and overall efficacy. Understanding these pathways is crucial for optimizing the compound’s therapeutic potential and minimizing potential side effects.
Transport and Distribution
The transport and distribution of this compound within cells and tissues are important factors that influence its activity and efficacy. The compound may interact with transporters and binding proteins that facilitate its uptake and distribution . Additionally, its localization and accumulation within specific tissues can impact its therapeutic effects. For example, in the brain, the compound’s ability to cross the blood-brain barrier and reach target neurons is critical for its efficacy in treating neurodegenerative diseases. Understanding the transport and distribution mechanisms of this compound can aid in the development of targeted therapies.
Subcellular Localization
The subcellular localization of this compound can influence its activity and function. The compound may be directed to specific compartments or organelles within cells through targeting signals or post-translational modifications . For instance, its localization to the synaptic cleft in neurons can enhance cholinergic signaling by inhibiting acetylcholine esterase. Additionally, its accumulation in the nucleus or mitochondria may impact gene expression and cellular metabolism. Understanding the subcellular localization of this compound can provide insights into its mechanism of action and potential therapeutic applications.
準備方法
Synthetic Routes and Reaction Conditions
The synthesis of N-(1-benzyl-2-oxo-1,2,3,4-tetrahydroquinolin-6-yl)butanamide typically involves multi-step reactions starting from commercially available starting materials. One common synthetic route includes the following steps:
Formation of the Quinoline Core: The quinoline core can be synthesized through the Pfitzinger reaction, which involves the condensation of an anthranilic acid derivative with a ketone under acidic conditions.
Introduction of the Benzyl Group: The benzyl group can be introduced through a Friedel-Crafts alkylation reaction, where benzyl chloride reacts with the quinoline core in the presence of a Lewis acid catalyst such as aluminum chloride.
Formation of the Butanamide Moiety: The final step involves the acylation of the quinoline derivative with butanoyl chloride in the presence of a base such as pyridine to form the butanamide moiety.
Industrial Production Methods
Industrial production of this compound may involve optimization of the above synthetic routes to improve yield and reduce costs. This can include the use of continuous flow reactors, green chemistry principles, and alternative solvents to enhance the efficiency and sustainability of the production process.
化学反応の分析
Types of Reactions
N-(1-benzyl-2-oxo-1,2,3,4-tetrahydroquinolin-6-yl)butanamide can undergo various chemical reactions, including:
Oxidation: The compound can be oxidized using oxidizing agents such as potassium permanganate or chromium trioxide to form quinoline N-oxides.
Reduction: Reduction reactions can be carried out using reducing agents like sodium borohydride or lithium aluminum hydride to convert the carbonyl group to an alcohol.
Substitution: The compound can undergo nucleophilic substitution reactions, where nucleophiles such as amines or thiols replace the benzyl group.
Common Reagents and Conditions
Oxidation: Potassium permanganate in acidic medium.
Reduction: Sodium borohydride in methanol.
Substitution: Ammonia or thiols in the presence of a base such as sodium hydroxide.
Major Products
Oxidation: Quinoline N-oxides.
Reduction: Alcohol derivatives.
Substitution: Amino or thiol-substituted quinoline derivatives.
科学的研究の応用
Chemistry: Used as a building block for the synthesis of more complex quinoline derivatives.
Biology: Investigated for its potential as an enzyme inhibitor or receptor modulator.
Medicine: Explored for its potential therapeutic effects, including anti-inflammatory, anticancer, and antimicrobial activities.
Industry: Utilized in the development of new materials and as a precursor for the synthesis of dyes and pigments.
類似化合物との比較
N-(1-benzyl-2-oxo-1,2,3,4-tetrahydroquinolin-6-yl)butanamide can be compared with other quinoline derivatives such as:
Quinoline-2,4-dione: Known for its biological and pharmacological activities.
4-Hydroxyquinoline: Used in the synthesis of various pharmaceuticals.
Quinolone antibiotics: Widely used as antibacterial agents.
The uniqueness of this compound lies in its specific structure, which imparts distinct biological activities and potential therapeutic applications.
特性
IUPAC Name |
N-(1-benzyl-2-oxo-3,4-dihydroquinolin-6-yl)butanamide |
Source
|
---|---|---|
Details | Computed by Lexichem TK 2.7.0 (PubChem release 2021.05.07) | |
Source | PubChem | |
URL | https://pubchem.ncbi.nlm.nih.gov | |
Description | Data deposited in or computed by PubChem | |
InChI |
InChI=1S/C20H22N2O2/c1-2-6-19(23)21-17-10-11-18-16(13-17)9-12-20(24)22(18)14-15-7-4-3-5-8-15/h3-5,7-8,10-11,13H,2,6,9,12,14H2,1H3,(H,21,23) |
Source
|
Details | Computed by InChI 1.0.6 (PubChem release 2021.05.07) | |
Source | PubChem | |
URL | https://pubchem.ncbi.nlm.nih.gov | |
Description | Data deposited in or computed by PubChem | |
InChI Key |
DBXVCZRTXZCZAP-UHFFFAOYSA-N |
Source
|
Details | Computed by InChI 1.0.6 (PubChem release 2021.05.07) | |
Source | PubChem | |
URL | https://pubchem.ncbi.nlm.nih.gov | |
Description | Data deposited in or computed by PubChem | |
Canonical SMILES |
CCCC(=O)NC1=CC2=C(C=C1)N(C(=O)CC2)CC3=CC=CC=C3 |
Source
|
Details | Computed by OEChem 2.3.0 (PubChem release 2021.05.07) | |
Source | PubChem | |
URL | https://pubchem.ncbi.nlm.nih.gov | |
Description | Data deposited in or computed by PubChem | |
Molecular Formula |
C20H22N2O2 |
Source
|
Details | Computed by PubChem 2.1 (PubChem release 2021.05.07) | |
Source | PubChem | |
URL | https://pubchem.ncbi.nlm.nih.gov | |
Description | Data deposited in or computed by PubChem | |
Molecular Weight |
322.4 g/mol |
Source
|
Details | Computed by PubChem 2.1 (PubChem release 2021.05.07) | |
Source | PubChem | |
URL | https://pubchem.ncbi.nlm.nih.gov | |
Description | Data deposited in or computed by PubChem | |
試験管内研究製品の免責事項と情報
BenchChemで提示されるすべての記事および製品情報は、情報提供を目的としています。BenchChemで購入可能な製品は、生体外研究のために特別に設計されています。生体外研究は、ラテン語の "in glass" に由来し、生物体の外で行われる実験を指します。これらの製品は医薬品または薬として分類されておらず、FDAから任何の医療状態、病気、または疾患の予防、治療、または治癒のために承認されていません。これらの製品を人間または動物に体内に導入する形態は、法律により厳格に禁止されています。これらのガイドラインに従うことは、研究と実験において法的および倫理的な基準の遵守を確実にするために重要です。