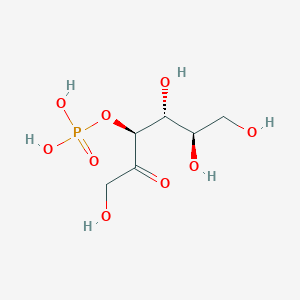
Fructose 3-phosphate
概要
説明
Fructose 3-phosphate is a sugar phosphate derived from fructose. It is one of the several phosphorylated derivatives of fructose that play crucial roles in various metabolic pathways, including glycolysis, gluconeogenesis, and the pentose phosphate pathway . This compound is particularly significant in the context of non-enzymatic glycation processes, which are implicated in diabetic complications .
準備方法
Synthetic Routes and Reaction Conditions: . This enzyme catalyzes the transfer of a phosphate group from adenosine triphosphate to the third carbon of fructose, resulting in the formation of fructose 3-phosphate.
Industrial Production Methods: While there is limited information on the large-scale industrial production of this compound, it is typically produced in research settings using enzymatic methods. The enzyme fructose-3-phosphokinase is purified from sources such as human erythrocytes and other mammalian tissues .
化学反応の分析
Types of Reactions: Fructose 3-phosphate undergoes various chemical reactions, including:
Phosphorylation: It can be further phosphorylated to form other sugar phosphates involved in metabolic pathways.
Common Reagents and Conditions:
Oxidation: Common oxidizing agents include reactive oxygen species and other oxidative stress-related compounds.
Phosphorylation: Enzymes such as fructose-3-phosphokinase and adenosine triphosphate are commonly used in phosphorylation reactions.
Major Products Formed:
Advanced Glycation End Products: These are formed through the oxidation of this compound and are implicated in various pathological conditions.
科学的研究の応用
Biochemical Role in Diabetes
F3P has been identified as a key metabolite in the lenses of diabetic rats, where it is linked to the development of diabetic complications. Research indicates that F3P acts as a protein glycosylating agent and enzyme inactivator. Its accumulation in diabetic conditions contributes to increased levels of advanced glycation end products (AGEs), which are implicated in various diabetic complications, such as cataract formation and lens opacity .
Case Study: Inhibition of Glycation
A study investigated the effects of F3P on fructosamine-3-kinase (FN3K), an enzyme responsible for phosphorylating glycated proteins. The results showed that F3P significantly inhibited FN3K activity, leading to decreased production of fructoselysine-3-phosphate (FL3P) in the diabetic lens. This inhibition was observed at concentrations similar to those found in diabetic conditions, suggesting that elevated F3P levels may exacerbate protein glycation and contribute to lens complications .
Potential Therapeutic Applications
Given its role in glycation and diabetes, F3P is being explored for potential therapeutic applications. Reducing F3P levels may enhance FN3K activity, thereby decreasing the accumulation of AGEs and improving outcomes for diabetic patients.
Research Insights
- Enzyme Activity Modulation : Studies have shown that manipulating F3P concentrations can modulate enzyme activities involved in glycation processes. For instance, reducing F3P levels in diabetic models led to increased FL3P production, which could mitigate some deleterious effects of glycation .
- Diabetic Complications : The hydrolysis of F3P produces 3-deoxyglucosone, another potent glycosylating agent. Understanding the balance between these metabolites could lead to strategies for managing diabetes-related complications .
Impact on Protein Functionality
F3P's ability to modify proteins through glycosylation has implications for protein functionality and stability. The phosphorylation by F3P can alter protein interactions and enzymatic activities, potentially leading to cellular dysfunction.
Table: Effects of this compound on Protein Glycation
Study | Findings | Implications |
---|---|---|
Szwergold et al. (2003) | F3P inhibited FN3K activity by 50-60% | Suggests a mechanism for increased protein glycation in diabetes |
Delpierre et al. (2000) | Erythrocytes convert fructose to F3P efficiently | Indicates potential metabolic pathways involving F3P |
Science Journal (1997) | Identified role of F3P in lens glycation processes | Highlights importance in understanding diabetic eye diseases |
Future Directions and Research Needs
Further research is essential to fully elucidate the mechanisms through which F3P influences cellular processes and its potential as a therapeutic target. Key areas for future exploration include:
- Mechanistic Studies : Understanding how F3P interacts with various enzymes and proteins.
- Clinical Trials : Evaluating the efficacy of interventions aimed at reducing F3P levels in diabetic patients.
- Broader Implications : Investigating the role of F3P in other metabolic disorders beyond diabetes.
作用機序
Fructose 3-phosphate exerts its effects primarily through its involvement in metabolic pathways and glycation processes. It acts as a substrate for enzymes such as fructose-3-phosphokinase, leading to the formation of phosphorylated intermediates that participate in glycolysis and other metabolic pathways . Additionally, its oxidation leads to the formation of advanced glycation end products, which are implicated in diabetic complications .
類似化合物との比較
- Fructose 1-phosphate
- Fructose 2-phosphate
- Fructose 6-phosphate
- Fructose 1,6-bisphosphate
- Fructose 2,6-bisphosphate
Comparison: Fructose 3-phosphate is unique in its specific role in non-enzymatic glycation processes and its formation through the action of fructose-3-phosphokinase . Unlike other fructose phosphates, which are primarily involved in glycolysis and gluconeogenesis, this compound is particularly significant in the context of diabetic complications and the formation of advanced glycation end products .
生物活性
Fructose 3-phosphate (Fru-3-P) is a phosphorylated derivative of fructose that plays a significant role in various metabolic pathways, particularly in carbohydrate metabolism. This article explores the biological activity of Fru-3-P, highlighting its biochemical functions, interactions, and implications in health and disease.
Overview of this compound
This compound is an intermediate in the metabolism of fructose, primarily involved in the conversion of fructose to glucose and other metabolites. It is produced from fructose through phosphorylation by fructokinase, leading to its involvement in glycolysis and gluconeogenesis. The compound serves as a substrate for various enzymatic reactions, influencing metabolic pathways crucial for energy production and cellular function.
Biochemical Pathways Involving Fru-3-P
-
Metabolic Conversion :
- Fru-3-P can be converted into glyceraldehyde-3-phosphate (GAP) and dihydroxyacetone phosphate (DHAP) through the action of aldolase enzymes, which are critical in both glycolysis and gluconeogenesis.
- It also participates in the polyol pathway, where it can be involved in the formation of advanced glycation end-products (AGEs), which are implicated in diabetic complications.
-
Role in Glycation :
- Research indicates that fructose accelerates the production of AGEs more than glucose due to its higher reactivity as a reducing sugar. This process is mediated by dicarbonyl compounds such as 3-deoxyglucosone (3DG), which can form from Fru-3-P and contribute to oxidative stress and cellular damage .
1. Glycation and Protein Modification
Fructose, through its metabolite Fru-3-P, has been shown to enhance protein cross-linking and polymerization, leading to increased fluorescence intensity in glycated proteins compared to glucose. This suggests a more pronounced effect on protein structure and function due to higher reactive intermediates formed during fructose metabolism .
2. Lipid Metabolism
Computational models have demonstrated that fructose consumption can lead to dyslipidemia by promoting triglyceride accumulation in the liver. The model indicated that changes in carbohydrate input significantly affect lipid profiles, with fructose being more potent than glucose . This highlights the role of Fru-3-P in lipid metabolism and its potential implications for metabolic disorders.
3. Impact on Insulin Resistance
Studies have shown that high levels of dietary fructose can contribute to insulin resistance, a precursor for type 2 diabetes. The metabolic pathways involving Fru-3-P may influence insulin signaling and glucose homeostasis, suggesting that excessive fructose intake could exacerbate metabolic syndromes .
Case Study 1: Fructose-Induced Hepatic Steatosis
A study involving Chrebp knockout mice demonstrated that high-fructose diets led to severe hepatic lipogenesis and steatosis. The absence of Chrebp exacerbated these effects, indicating that Fru-3-P plays a crucial role in regulating lipid metabolism under conditions of excess fructose intake .
Case Study 2: Diabetic Complications
Research has illustrated that individuals with high fructose consumption exhibit increased markers of oxidative stress and AGEs formation. The involvement of Fru-3-P in these pathways suggests that managing dietary fructose may be essential for preventing complications associated with diabetes .
特性
IUPAC Name |
[(3S,4R,5R)-1,4,5,6-tetrahydroxy-2-oxohexan-3-yl] dihydrogen phosphate | |
---|---|---|
Source | PubChem | |
URL | https://pubchem.ncbi.nlm.nih.gov | |
Description | Data deposited in or computed by PubChem | |
InChI |
InChI=1S/C6H13O9P/c7-1-3(9)5(11)6(4(10)2-8)15-16(12,13)14/h3,5-9,11H,1-2H2,(H2,12,13,14)/t3-,5-,6-/m1/s1 | |
Source | PubChem | |
URL | https://pubchem.ncbi.nlm.nih.gov | |
Description | Data deposited in or computed by PubChem | |
InChI Key |
NFKVQPCCMLVBGQ-UYFOZJQFSA-N | |
Source | PubChem | |
URL | https://pubchem.ncbi.nlm.nih.gov | |
Description | Data deposited in or computed by PubChem | |
Canonical SMILES |
C(C(C(C(C(=O)CO)OP(=O)(O)O)O)O)O | |
Source | PubChem | |
URL | https://pubchem.ncbi.nlm.nih.gov | |
Description | Data deposited in or computed by PubChem | |
Isomeric SMILES |
C([C@H]([C@H]([C@@H](C(=O)CO)OP(=O)(O)O)O)O)O | |
Source | PubChem | |
URL | https://pubchem.ncbi.nlm.nih.gov | |
Description | Data deposited in or computed by PubChem | |
Molecular Formula |
C6H13O9P | |
Source | PubChem | |
URL | https://pubchem.ncbi.nlm.nih.gov | |
Description | Data deposited in or computed by PubChem | |
DSSTOX Substance ID |
DTXSID90925470 | |
Record name | 3-O-Phosphonohex-2-ulose | |
Source | EPA DSSTox | |
URL | https://comptox.epa.gov/dashboard/DTXSID90925470 | |
Description | DSSTox provides a high quality public chemistry resource for supporting improved predictive toxicology. | |
Molecular Weight |
260.14 g/mol | |
Source | PubChem | |
URL | https://pubchem.ncbi.nlm.nih.gov | |
Description | Data deposited in or computed by PubChem | |
CAS No. |
126247-74-3 | |
Record name | Fructose 3-phosphate | |
Source | ChemIDplus | |
URL | https://pubchem.ncbi.nlm.nih.gov/substance/?source=chemidplus&sourceid=0126247743 | |
Description | ChemIDplus is a free, web search system that provides access to the structure and nomenclature authority files used for the identification of chemical substances cited in National Library of Medicine (NLM) databases, including the TOXNET system. | |
Record name | 3-O-Phosphonohex-2-ulose | |
Source | EPA DSSTox | |
URL | https://comptox.epa.gov/dashboard/DTXSID90925470 | |
Description | DSSTox provides a high quality public chemistry resource for supporting improved predictive toxicology. | |
Retrosynthesis Analysis
AI-Powered Synthesis Planning: Our tool employs the Template_relevance Pistachio, Template_relevance Bkms_metabolic, Template_relevance Pistachio_ringbreaker, Template_relevance Reaxys, Template_relevance Reaxys_biocatalysis model, leveraging a vast database of chemical reactions to predict feasible synthetic routes.
One-Step Synthesis Focus: Specifically designed for one-step synthesis, it provides concise and direct routes for your target compounds, streamlining the synthesis process.
Accurate Predictions: Utilizing the extensive PISTACHIO, BKMS_METABOLIC, PISTACHIO_RINGBREAKER, REAXYS, REAXYS_BIOCATALYSIS database, our tool offers high-accuracy predictions, reflecting the latest in chemical research and data.
Strategy Settings
Precursor scoring | Relevance Heuristic |
---|---|
Min. plausibility | 0.01 |
Model | Template_relevance |
Template Set | Pistachio/Bkms_metabolic/Pistachio_ringbreaker/Reaxys/Reaxys_biocatalysis |
Top-N result to add to graph | 6 |
Feasible Synthetic Routes
試験管内研究製品の免責事項と情報
BenchChemで提示されるすべての記事および製品情報は、情報提供を目的としています。BenchChemで購入可能な製品は、生体外研究のために特別に設計されています。生体外研究は、ラテン語の "in glass" に由来し、生物体の外で行われる実験を指します。これらの製品は医薬品または薬として分類されておらず、FDAから任何の医療状態、病気、または疾患の予防、治療、または治癒のために承認されていません。これらの製品を人間または動物に体内に導入する形態は、法律により厳格に禁止されています。これらのガイドラインに従うことは、研究と実験において法的および倫理的な基準の遵守を確実にするために重要です。