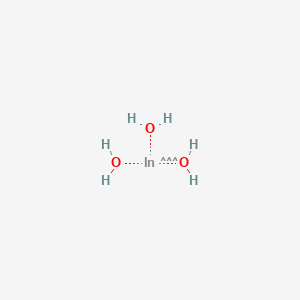
水酸化インジウム(III)
概要
説明
Indium(III) hydroxide is the chemical compound with the formula In(OH)3. It is sometimes found as the rare mineral dzhalindite . Its prime use is as a precursor to indium(III) oxide, In2O3 . Indium(III) hydroxide is amphoteric, like gallium(III) hydroxide (Ga(OH)3) and aluminium hydroxide (Al(OH)3), but is much less acidic than gallium hydroxide (Ga(OH)3), having a lower solubility in alkaline solutions than in acid solutions .
Synthesis Analysis
Indium hydroxides were prepared by the mixing of aqueous indium nitrate solution with sodium or ammonium hydroxide solutions under various conditions . Indium hydroxide nanostructures were synthesized by sol-gel and hydrothermal processes from indium acetate and sodium hydroxide as precursors and polyvinyl alcohol, polyvinyl pyrrolidone or polydimethylsiloxane as stabilizers .Molecular Structure Analysis
Indium(III) hydroxide has a cubic structure, space group Im3, a distorted ReO3 structure . The electrostatic stability of nanoparticles is carried out through zeta potential measurement .Chemical Reactions Analysis
Neutralizing a solution containing an In3+ salt such as indium nitrate (In(NO3)3) or a solution of indium trichloride (InCl3) gives a white precipitate that on aging forms indium(III) hydroxide . A thermal decomposition of freshly prepared In(OH)3 shows the first step is the conversion of In(OH)3·xH2O to cubic indium(III) hydroxide .Physical and Chemical Properties Analysis
Indium hydroxide is a white solid with a density of 4.38 g/cm3 . It has a melting point of 150 °C (302 °F; 423 K) and is insoluble in water . The refractive index (nD) is 1.725 . The molar mass is 165.8404 g/mol .科学的研究の応用
抗菌、抗真菌、抗ウイルス可能性:
3ナノ粒子合成と産業
インジウム(III)錯体は、実験室以外でも用途が見出されています:
カルコゲニドナノ粒子の制御合成:要約すると、水酸化インジウム(III)とその錯体は、生物学的、医学的、合成的、産業的ドメインにわたる幅広い用途を提供しています。その汎用性と独自の特性により、研究とイノベーションが促進され続けています。
Ajiboye, T. O., Amao, I. O., Adeyemi, W. J., Babalola, S. O., Akinsuyi, O. S., Ogunrombi, M. O., Ogunlaja, A. S., & Mhlanga, S. D. (2024). インジウム(III)錯体の医学および生物学的用途の概要Chemistry Africa, 7, 1729–1748. Frost, C. G., & Hartley, R. C. (2004). インジウム(III)錯体: 有機触媒としての用途Journal of Chemical Sciences, 116(5), 297–303. Masikane, T. M., & Revaprasadu, N. (2020). インジウム(III)錯体: 有機触媒、カルコゲニドナノ粒子の前駆体、および産業における出発物質としての用途。 Journal of Chemical Sciences, 136(4), 1–10.
作用機序
Target of Action
Indium(III) hydroxide, also known as In(OH)3, primarily targets a wide range of biological and medical applications . The properties of these complexes depend on the primary ligand that was used for their syntheses .
Mode of Action
The specific mechanism of action of indium(III) complexes in antimicrobial therapy can vary depending on the type of microorganism being targeted . They have shown antibacterial activity against both Gram-negative and Gram-positive bacteria .
Biochemical Pathways
The formation of the indium complexes is achieved by reacting indium salt with the dithiocarbamate ligands . These complexes are versatile species that emit Auger electrons, making them a choice for a wide range of biological and medical applications .
Pharmacokinetics
The properties of indium(iii) complexes depend on the primary ligand that was used for their syntheses . This suggests that the absorption, distribution, metabolism, and excretion (ADME) properties of indium(III) hydroxide could be influenced by the ligands it is complexed with.
Result of Action
Indium(III) complexes have been extensively studied for their antimicrobial activities against various microorganisms, including bacteria, fungi, viruses, and parasites . Other biological applications of the complexes such as anticancer, bioimaging, radiopharmaceutical, photodynamic chemotherapy, antioxidants, and optical limiting applications of these indium(III) complexes are comprehensively reviewed .
Action Environment
The reactions are often carried out in the environment of alkyl halides, epoxides, transition metals and imines which are electrophiles . The environment can influence the action, efficacy, and stability of indium(III) hydroxide. For instance, at 10 MPa pressure and 250-400 °C, indium(III) hydroxide converts to indium oxide hydroxide (InO(OH)), which has a distorted rutile structure .
Safety and Hazards
将来の方向性
The indium oxides, c-In2O3, h-In2O3, InOOH and In(OH)3, constitute an important class of wide band gap semiconductors . Synthesis of any indium oxide phase involves maneuvering in a complex matrix of process parameters, and some phases are only obtained through controlled phase transformations . This insight is used to develop procedures for scalable continuous flow solvothermal synthesis .
生化学分析
Biochemical Properties
Indium(III) complexes are versatile species that emit Auger electrons which made them a choice for a wide range of biological and medical applications . The properties of these complexes depend on the primary ligand that was used for their syntheses . In biological systems, metal ions often form complexes with various ligands, including proteins, enzymes, and other biomolecules . The stability of these metal complexes is crucial for their functionality and reactivity within biological processes .
Cellular Effects
Indium(III) complexes have shown cytotoxic effects against several human tumor cells . For instance, an indium(III) complex of the [In(L)3] type, where HL = 5-hydroxyflavone (primuletin), has been reported to exhibit modest cytotoxic activity .
Molecular Mechanism
At 10 MPa pressure and 250-400 °C, indium(III) hydroxide converts to indium oxide hydroxide (InO(OH)), which has a distorted rutile structure . Rapid decompression of samples of indium(III) hydroxide compressed at 34 GPa causes decomposition, yielding some indium metal .
Temporal Effects in Laboratory Settings
A thermal decomposition of freshly prepared In(OH)3 shows the first step is the conversion of In(OH)3·xH2O to cubic indium(III) hydroxide . At 10 MPa pressure and 250-400 °C, indium(III) hydroxide converts to indium oxide hydroxide (InO(OH)), which has a distorted rutile structure .
特性
InChI |
InChI=1S/In.3H2O/h;3*1H2 | |
---|---|---|
Source | PubChem | |
URL | https://pubchem.ncbi.nlm.nih.gov | |
Description | Data deposited in or computed by PubChem | |
InChI Key |
ZMFWDTJZHRDHNW-UHFFFAOYSA-N | |
Source | PubChem | |
URL | https://pubchem.ncbi.nlm.nih.gov | |
Description | Data deposited in or computed by PubChem | |
Canonical SMILES |
O.O.O.[In] | |
Source | PubChem | |
URL | https://pubchem.ncbi.nlm.nih.gov | |
Description | Data deposited in or computed by PubChem | |
Molecular Formula |
H6InO3 | |
Source | PubChem | |
URL | https://pubchem.ncbi.nlm.nih.gov | |
Description | Data deposited in or computed by PubChem | |
Molecular Weight |
168.864 g/mol | |
Source | PubChem | |
URL | https://pubchem.ncbi.nlm.nih.gov | |
Description | Data deposited in or computed by PubChem | |
CAS No. |
20661-21-6 | |
Record name | Indium hydroxide | |
Source | DTP/NCI | |
URL | https://dtp.cancer.gov/dtpstandard/servlet/dwindex?searchtype=NSC&outputformat=html&searchlist=127173 | |
Description | The NCI Development Therapeutics Program (DTP) provides services and resources to the academic and private-sector research communities worldwide to facilitate the discovery and development of new cancer therapeutic agents. | |
Explanation | Unless otherwise indicated, all text within NCI products is free of copyright and may be reused without our permission. Credit the National Cancer Institute as the source. | |
Synthesis routes and methods I
Procedure details
Synthesis routes and methods II
Procedure details
Retrosynthesis Analysis
AI-Powered Synthesis Planning: Our tool employs the Template_relevance Pistachio, Template_relevance Bkms_metabolic, Template_relevance Pistachio_ringbreaker, Template_relevance Reaxys, Template_relevance Reaxys_biocatalysis model, leveraging a vast database of chemical reactions to predict feasible synthetic routes.
One-Step Synthesis Focus: Specifically designed for one-step synthesis, it provides concise and direct routes for your target compounds, streamlining the synthesis process.
Accurate Predictions: Utilizing the extensive PISTACHIO, BKMS_METABOLIC, PISTACHIO_RINGBREAKER, REAXYS, REAXYS_BIOCATALYSIS database, our tool offers high-accuracy predictions, reflecting the latest in chemical research and data.
Strategy Settings
Precursor scoring | Relevance Heuristic |
---|---|
Min. plausibility | 0.01 |
Model | Template_relevance |
Template Set | Pistachio/Bkms_metabolic/Pistachio_ringbreaker/Reaxys/Reaxys_biocatalysis |
Top-N result to add to graph | 6 |
Feasible Synthetic Routes
Q1: What is the molecular formula and weight of Indium(III) hydroxide?
A1: The molecular formula of Indium(III) hydroxide is In(OH)₃, and its molecular weight is 165.82 g/mol.
Q2: What crystal structures can Indium(III) hydroxide adopt?
A2: Indium(III) hydroxide can crystallize in both cubic and orthorhombic forms. [, , ] For example, pure cubic In(OH)₃ nanocrystals were synthesized through a controlled aqueous solvothermal route. [] Researchers have also synthesized orthorhombic In(OH)₃ nanocubes using a simple amino acid-assisted hydrothermal process. []
Q3: What spectroscopic techniques are used to characterize Indium(III) hydroxide?
A3: Various techniques are employed, including:
- X-ray diffraction (XRD): Used to determine the crystal structure and phase purity of In(OH)₃. [, , , ]
- Scanning electron microscopy (SEM) and Transmission electron microscopy (TEM): Provide information about the morphology and size of In(OH)₃ particles, such as nanocubes, nanorods, and flower-like hierarchical structures. [, , , ]
- Infrared (IR) spectroscopy: Identifies functional groups, including the characteristic O-H stretching and bending vibrations in In(OH)₃. [, ]
- X-ray photoelectron spectroscopy (XPS): Analyzes the elemental composition and chemical states of Indium and Oxygen in In(OH)₃. []
- Nuclear magnetic resonance (NMR) spectroscopy: Provides insights into the local structure and proton distribution in In(OH)₃. []
Q4: What are the key applications of Indium(III) hydroxide?
A4: Indium(III) hydroxide finds applications in various fields, including:
- Precursor for Indium Oxide (In₂O₃): It serves as a precursor for the synthesis of In₂O₃, a transparent conducting oxide with applications in optoelectronics and gas sensors. [, , ]
- Photocatalysis: In(OH)₃ nanostructures have shown potential as photocatalysts for the degradation of organic pollutants like Rhodamine B dye under UV irradiation. [, ]
- Gas Sensing: In(OH)₃-based composites, like In(OH)₃/Co(OH)₂/rGO, have demonstrated excellent gas sensing properties towards NOx at room temperature, highlighting their potential for environmental monitoring. []
Q5: How does the morphology of Indium(III) hydroxide influence its properties?
A5: The morphology of In(OH)₃ significantly impacts its properties:
- Surface Area: Nanostructures, such as nanocubes or flower-like hierarchical structures, offer a high surface area, providing more active sites for photocatalytic reactions or gas adsorption. [, ]
- Charge Transport: The interconnected nature of certain morphologies, like nanorods in a composite, can enhance charge transport, improving gas sensing performance. []
Q6: What factors influence the conversion of Indium(III) hydroxide to Indium oxide?
A6: Several factors affect the transformation:
- Temperature: Calcination of In(OH)₃ at elevated temperatures leads to its decomposition into In₂O₃. [, ]
- Atmosphere: The atmosphere during heat treatment can influence the phase and properties of the resulting In₂O₃. []
Q7: How is Indium(III) hydroxide used in the synthesis of other materials?
A7: In(OH)₃ acts as a precursor and can be combined with other metal ions to synthesize mixed-metal oxides or hydroxides with tailored properties. [] For example, it can be used to create La(OH)₃ nanosheet-supported CoPt nanoparticles, which act as effective catalysts for hydrogen production. []
試験管内研究製品の免責事項と情報
BenchChemで提示されるすべての記事および製品情報は、情報提供を目的としています。BenchChemで購入可能な製品は、生体外研究のために特別に設計されています。生体外研究は、ラテン語の "in glass" に由来し、生物体の外で行われる実験を指します。これらの製品は医薬品または薬として分類されておらず、FDAから任何の医療状態、病気、または疾患の予防、治療、または治癒のために承認されていません。これらの製品を人間または動物に体内に導入する形態は、法律により厳格に禁止されています。これらのガイドラインに従うことは、研究と実験において法的および倫理的な基準の遵守を確実にするために重要です。