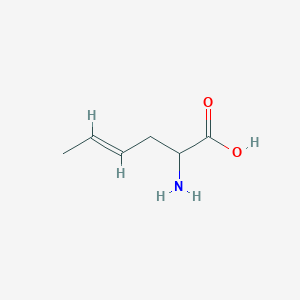
2-Amino-4-hexenoic acid
概要
説明
2-Amino-4-hexenoic acid is an organic compound that belongs to the class of amino acids It is characterized by the presence of an amino group (-NH2) attached to the fourth carbon of a hexenoic acid chain
準備方法
Synthetic Routes and Reaction Conditions: The synthesis of 2-amino-4-hexenoic acid can be achieved through several methods. One common approach involves the use of succinimide as a starting material. The process includes the following steps:
Formation of Intermediate: Succinimide is first converted into an intermediate compound through a series of chemical reactions.
Amination: The intermediate is then subjected to amination reactions to introduce the amino group.
Final Conversion: The final step involves the conversion of the aminated intermediate into this compound under controlled conditions.
Industrial Production Methods: Industrial production of this compound typically involves large-scale synthesis using optimized reaction conditions to ensure high yield and purity. The process may include the use of catalysts and specific reaction environments to facilitate the conversion of starting materials into the desired product .
化学反応の分析
Types of Reactions: 2-Amino-4-hexenoic acid undergoes various chemical reactions, including:
Oxidation: The compound can be oxidized to form corresponding oxo derivatives.
Reduction: Reduction reactions can convert the double bond into a single bond, resulting in saturated amino acids.
Substitution: The amino group can participate in substitution reactions, leading to the formation of various derivatives.
Common Reagents and Conditions:
Oxidation: Common oxidizing agents include potassium permanganate and hydrogen peroxide.
Reduction: Reducing agents such as sodium borohydride and lithium aluminum hydride are often used.
Substitution: Substitution reactions may involve reagents like halogens and alkylating agents.
Major Products: The major products formed from these reactions include oxo derivatives, saturated amino acids, and various substituted amino acids, depending on the specific reaction conditions and reagents used .
科学的研究の応用
Chemistry
2-Amino-4-hexenoic acid is utilized as a building block for synthesizing more complex molecules. It acts as a reagent in various chemical reactions, contributing to the development of new compounds in organic chemistry.
Biology
In biological research, this compound is studied for its role as an amino acid analogue . Specifically, it interacts with methionyl tRNA synthetase, competing with methionine during protein synthesis. This interaction can disrupt normal protein structure and function, making it a valuable tool for studying protein engineering and metabolic pathways.
Medicine
Current research is exploring the potential therapeutic applications of this compound, particularly as an antiepileptic agent . Preliminary studies suggest that it may influence neurotransmitter systems and offer neuroprotective effects.
Industrial Applications
The compound finds use in the production of pharmaceuticals and other industrial chemicals. Its properties allow it to be integrated into formulations that require specific biochemical interactions or enhancements.
Case Study 1: Protein Engineering
In a study examining the effects of this compound on protein synthesis, researchers found that its incorporation into proteins could lead to altered functionality and stability. This was demonstrated through experiments where bacterial cells were treated with varying concentrations of the compound, resulting in significant changes in protein expression profiles.
Case Study 2: Antiepileptic Research
A recent investigation into the pharmacological properties of this compound indicated its potential as an antiepileptic drug. In vitro studies showed that the compound could modulate synaptic transmission and reduce seizure activity in animal models, highlighting its therapeutic promise.
Comparative Data Table
Application Area | Description | Key Findings |
---|---|---|
Chemistry | Building block for complex molecules | Essential for synthesizing various derivatives |
Biology | Amino acid analogue affecting protein synthesis | Competes with methionine, altering protein function |
Medicine | Potential antiepileptic agent | Modulates neurotransmission; reduces seizure activity |
Industry | Used in pharmaceuticals production | Enhances biochemical formulations |
作用機序
The mechanism of action of 2-amino-4-hexenoic acid involves its interaction with specific molecular targets and pathways:
Molecular Targets: The compound targets enzymes and receptors involved in amino acid metabolism and neurotransmission.
類似化合物との比較
2-Amino-4-hexenoic acid can be compared with other similar compounds, such as:
2-Amino-5-chloro-4-hydroxy-5-hexenoic acid: This compound contains a chlorine and hydroxyl group, making it distinct in terms of reactivity and applications.
2-Amino-4,5-hexadienoic acid: This compound has an additional double bond, which affects its chemical properties and reactivity.
2-Amino-5-hexynoic acid: The presence of a triple bond in this compound significantly alters its chemical behavior compared to this compound.
These comparisons highlight the unique structural features and reactivity of this compound, making it a valuable compound for various scientific and industrial applications.
生物活性
2-Amino-4-hexenoic acid (AHA) is a non-proteinogenic amino acid that has garnered attention for its unique structural properties and biological activities, particularly in the context of antibiotic biosynthesis. This article explores the biological activity of AHA, focusing on its role in the biosynthesis of ilamycins, a class of antibiotics, and its potential therapeutic applications.
Chemical Structure and Properties
This compound is characterized by the following chemical structure:
- Chemical Formula : C6H11NO2
- Molecular Weight : 115.16 g/mol
The presence of a double bond in its backbone contributes to its reactivity and biological function.
Role in Antibiotic Biosynthesis
AHA has been identified as a crucial building block in the biosynthesis of ilamycins, which are produced by the marine-derived Streptomyces atratus. Recent studies have demonstrated that AHA is incorporated into ilamycin structures through nonribosomal peptide synthetases (NRPSs), highlighting its significance in antibiotic production.
Table 1: Key Findings on AHA in Ilamycin Biosynthesis
The incorporation of AHA into ilamycins enhances their biological activity. The biosynthesis involves several enzymatic steps:
- Precursor Feeding : Mutant strains were fed synthesized AHA, restoring ilamycin production, confirming AHA's role as a precursor.
- Gene Knockout Studies : Deletion of specific genes involved in AHA metabolism resulted in significantly reduced titers of ilamycins, emphasizing the importance of AHA in the biosynthetic pathway.
Case Study 1: Anti-Tuberculosis Activity
In a study involving Mycobacterium tuberculosis, ilamycins containing AHA demonstrated remarkable potency. The engineered strains producing high levels of ilamycins showed an MIC value around 9.8 nM, indicating their potential as effective anti-tuberculosis agents .
Case Study 2: Toxicity Profile
Research has indicated that while AHA contributes to antibiotic efficacy, its metabolic products may have implications for toxicity. Studies on Wistar rats exposed to anti-tuberculosis drugs revealed alterations in oxidative stress markers and apoptosis-related gene expression, suggesting that compounds like AHA may play a role in both therapeutic effects and potential side effects .
特性
IUPAC Name |
(E)-2-aminohex-4-enoic acid | |
---|---|---|
Source | PubChem | |
URL | https://pubchem.ncbi.nlm.nih.gov | |
Description | Data deposited in or computed by PubChem | |
InChI |
InChI=1S/C6H11NO2/c1-2-3-4-5(7)6(8)9/h2-3,5H,4,7H2,1H3,(H,8,9)/b3-2+ | |
Source | PubChem | |
URL | https://pubchem.ncbi.nlm.nih.gov | |
Description | Data deposited in or computed by PubChem | |
InChI Key |
OPOBBDXDRHKTJF-NSCUHMNNSA-N | |
Source | PubChem | |
URL | https://pubchem.ncbi.nlm.nih.gov | |
Description | Data deposited in or computed by PubChem | |
Canonical SMILES |
CC=CCC(C(=O)O)N | |
Source | PubChem | |
URL | https://pubchem.ncbi.nlm.nih.gov | |
Description | Data deposited in or computed by PubChem | |
Isomeric SMILES |
C/C=C/CC(C(=O)O)N | |
Source | PubChem | |
URL | https://pubchem.ncbi.nlm.nih.gov | |
Description | Data deposited in or computed by PubChem | |
Molecular Formula |
C6H11NO2 | |
Source | PubChem | |
URL | https://pubchem.ncbi.nlm.nih.gov | |
Description | Data deposited in or computed by PubChem | |
Molecular Weight |
129.16 g/mol | |
Source | PubChem | |
URL | https://pubchem.ncbi.nlm.nih.gov | |
Description | Data deposited in or computed by PubChem | |
CAS No. |
28024-56-8 | |
Record name | 4-Hexonoic acid, 2-amino-, (+-)- | |
Source | ChemIDplus | |
URL | https://pubchem.ncbi.nlm.nih.gov/substance/?source=chemidplus&sourceid=0028024568 | |
Description | ChemIDplus is a free, web search system that provides access to the structure and nomenclature authority files used for the identification of chemical substances cited in National Library of Medicine (NLM) databases, including the TOXNET system. | |
Retrosynthesis Analysis
AI-Powered Synthesis Planning: Our tool employs the Template_relevance Pistachio, Template_relevance Bkms_metabolic, Template_relevance Pistachio_ringbreaker, Template_relevance Reaxys, Template_relevance Reaxys_biocatalysis model, leveraging a vast database of chemical reactions to predict feasible synthetic routes.
One-Step Synthesis Focus: Specifically designed for one-step synthesis, it provides concise and direct routes for your target compounds, streamlining the synthesis process.
Accurate Predictions: Utilizing the extensive PISTACHIO, BKMS_METABOLIC, PISTACHIO_RINGBREAKER, REAXYS, REAXYS_BIOCATALYSIS database, our tool offers high-accuracy predictions, reflecting the latest in chemical research and data.
Strategy Settings
Precursor scoring | Relevance Heuristic |
---|---|
Min. plausibility | 0.01 |
Model | Template_relevance |
Template Set | Pistachio/Bkms_metabolic/Pistachio_ringbreaker/Reaxys/Reaxys_biocatalysis |
Top-N result to add to graph | 6 |
Feasible Synthetic Routes
Q1: What is the mechanism by which 2-amino-4-hexenoic acid exerts its biological effects?
A1: this compound acts as an analogue of methionine, a naturally occurring amino acid essential for protein synthesis. Research suggests that its biological activity primarily stems from its interaction with methionyl tRNA synthetase (MetRS) []. This enzyme is responsible for attaching methionine to its specific transfer RNA (tRNA), a crucial step in protein synthesis. This compound exhibits high affinity for MetRS, effectively competing with methionine and becoming incorporated into proteins instead []. This substitution can disrupt protein structure and function, ultimately leading to the observed biological effects.
Q2: Can this compound be utilized in protein engineering applications?
A2: Yes, research demonstrates the successful incorporation of this compound into proteins in vivo []. Studies using E.coli as a model system show that it can substitute methionine with high efficiency, reaching nearly 85% replacement []. This capability opens up avenues for incorporating this unnatural amino acid into proteins, potentially introducing novel functionalities or serving as a tool for studying protein structure-function relationships.
Q3: Is there evidence of this compound being produced naturally?
A3: Interestingly, this compound is not merely a synthetic analogue. Research has identified it as a constituent of naturally occurring compounds called ilamycins [, ]. These molecules exhibit potent anti-tuberculosis activity, highlighting the potential of this unusual amino acid as a scaffold for developing new therapeutics. Understanding its biosynthetic pathway in ilamycin-producing organisms could provide valuable insights for future drug discovery efforts.
試験管内研究製品の免責事項と情報
BenchChemで提示されるすべての記事および製品情報は、情報提供を目的としています。BenchChemで購入可能な製品は、生体外研究のために特別に設計されています。生体外研究は、ラテン語の "in glass" に由来し、生物体の外で行われる実験を指します。これらの製品は医薬品または薬として分類されておらず、FDAから任何の医療状態、病気、または疾患の予防、治療、または治癒のために承認されていません。これらの製品を人間または動物に体内に導入する形態は、法律により厳格に禁止されています。これらのガイドラインに従うことは、研究と実験において法的および倫理的な基準の遵守を確実にするために重要です。