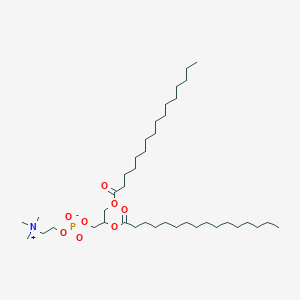
1,2-Dipalmitoylphosphatidylcholine
説明
Synthetic phospholipid used in liposomes and lipid bilayers to study biological membranes. It is also a major constituent of PULMONARY SURFACTANTS.
作用機序
Target of Action
1,2-Dipalmitoylphosphatidylcholine (DPPC) is a phospholipid and a lecithin, consisting of two C16 palmitic acid groups attached to a phosphatidylcholine head-group . It is the main constituent of pulmonary surfactants , which are surface-active materials produced by most air-breathing animals . The primary target of DPPC is the alveolar epithelium in the lungs .
Mode of Action
DPPC reduces the work of breathing and prevents alveolar collapse during breathing . Its structure includes both a hydrophilic “head” and hydrophobic “tails”, and it is this arrangement that makes it able to reduce the surface tension of the water layer . The choline radical constitutes the polar hydrophilic head; it is oriented towards and extends into the alveolar liquid . The palmitic acid (C16) chains form the nonpolar hydrophobic tails; these are oriented towards the outer side .
Biochemical Pathways
DPPC is biosynthesized via a de novo pathway, the last step of which is catalyzed by CDP-choline:cholinephosphotransferase (CPT) and two remodeling steps: a deacylation and a reacylation one, catalyzed by an acidic, Ca2±independent phospholipase A2 (ai PLA2) and a lyso-phosphatidylcholine acyltransferase (LPCAT), respectively .
Result of Action
The result of DPPC’s action is the reduction of surface tension in the alveoli, which facilitates gas exchange, prevents alveolar collapse, and reduces the work of breathing . It also plays an important role in the study of liposomes and human bilayers .
Action Environment
The action of DPPC can be influenced by environmental factors such as mechanical forces generated during tidal breathing . For example, a low magnitude, non-injurious static mode of mechanical stretch can induce phosphatidylcholine (PtdCho) biosynthesis and its remodeling to DPPC in lung epithelial cells . Thus, low magnitude stretch could contribute to protective mechanisms rather than to injurious ones .
生化学分析
Biochemical Properties
1,2-Dipalmitoylphosphatidylcholine is synthesized via a de novo pathway, the last step of which is catalyzed by CDP-choline:cholinephosphotransferase (CPT) and two remodeling steps: a deacylation and a reacylation one, catalyzed by an acidic, Ca2±independent phospholipase A2 and a lyso-phosphatidylcholine acyltransferase (LPCAT), respectively .
Cellular Effects
This compound has been shown to have effects on various types of cells and cellular processes. For example, it has been found that mild static mechanical stretch can promote the biosynthesis of phosphatidylcholine and its remodeling to this compound in lung epithelial cells .
Molecular Mechanism
The molecular mechanism of this compound involves interactions with various biomolecules. For instance, it is known to interact with cholesterol, and these interactions can influence the structure and physical properties of lipid bilayers .
Temporal Effects in Laboratory Settings
In laboratory settings, the effects of this compound can change over time. For example, an increase in phosphatidylcholine levels, especially among the this compound molecular species, was observed after 1 hour of static stretching in a study .
Metabolic Pathways
This compound is involved in various metabolic pathways. It is biosynthesized via a de novo pathway, the last step of which is catalyzed by CDP-choline:cholinephosphotransferase .
Subcellular Localization
The subcellular localization of this compound is primarily in the endoplasmic reticulum of type II pneumocytes, where it is synthesized
生物活性
1,2-Dipalmitoylphosphatidylcholine (DPPC) is a phospholipid that plays a crucial role in various biological processes, particularly in the respiratory system as a primary component of pulmonary surfactant. This article explores the biological activity of DPPC, focusing on its structural characteristics, physiological functions, and implications in medical applications.
Structural Characteristics
DPPC consists of two palmitic acid chains (C16:0) attached to a phosphatidylcholine head group. Its amphipathic nature allows it to form bilayers and micelles in aqueous environments, which is essential for its function in biological membranes.
Table 1: Structural Properties of DPPC
Property | Description |
---|---|
Molecular Formula | C36H70NO8P |
Molecular Weight | 634.87 g/mol |
Phase Transition Temperature | 41°C (gel to liquid crystalline phase) |
Hydrophilic Head Group | Phosphatidylcholine |
Hydrophobic Tails | Two palmitic acid chains |
Role in Pulmonary Surfactant
DPPC is the predominant component of pulmonary surfactant, which reduces surface tension in the alveoli, preventing collapse during exhalation and facilitating gas exchange. The presence of DPPC is vital for maintaining lung function and reducing the work of breathing.
- Surface Activity : DPPC significantly lowers the surface tension of the alveolar fluid, enhancing lung compliance and reducing the risk of atelectasis (lung collapse) .
- Biosynthesis : The synthesis of DPPC occurs primarily in type II alveolar cells through pathways involving cytidine diphosphate-choline (CDP-choline) .
Studies on Biological Activity
Several studies have investigated the biological activity of DPPC, revealing its effects on cellular interactions and drug delivery systems.
- Phagocytosis Reduction : A study demonstrated that incorporating DPPC into poly(lactic-co-glycolic acid) (PLGA) microspheres altered their surface chemistry, leading to reduced phagocytosis by lung macrophages. This suggests potential applications for controlled drug delivery to the lungs .
- Infection Facilitation : Research indicated that DPPC may enhance the infection of polarized cells by human adenovirus type C2. This effect is attributed to DPPC's ability to facilitate viral entry through the apical membrane .
- Degradation Kinetics : A study using atomic force microscopy analyzed the degradation kinetics of DPPC bilayers under enzymatic action from phospholipase A2, providing insights into its stability and interactions with other biomolecules .
Clinical Applications
DPPC has been extensively studied for its therapeutic applications, particularly in treating neonatal respiratory distress syndrome (RDS). Synthetic surfactants containing DPPC have shown improved clinical outcomes in preterm infants with RDS.
- Historical Context : Initial treatments using aerosolized DPPC alone were ineffective; however, subsequent formulations combining DPPC with other lipids have demonstrated significant benefits .
- Current Formulations : Modern surfactant therapies often include a mix of DPPC and other phospholipids and proteins to enhance efficacy .
科学的研究の応用
Biological Significance
DPPC is primarily known as a major component of pulmonary surfactant, which is essential for reducing surface tension in the lungs and preventing alveolar collapse during respiration. Its unique structure, featuring two palmitic acid chains and a phosphatidylcholine head group, allows it to interact effectively with air-liquid interfaces in the alveoli .
Pulmonary Surfactant
- Role in Respiratory Function : DPPC is critical in the formulation of surfactants used to treat respiratory distress syndrome (RDS) in neonates. It aids in reducing surface tension, thereby improving lung function and oxygenation in premature infants .
- Clinical Use : Synthetic surfactants containing DPPC have been developed since the 1960s, with significant improvements observed in clinical outcomes for infants suffering from RDS .
Research Applications
DPPC is extensively utilized in research settings, particularly in studies involving liposomes and cell membranes.
Liposome Formation
- Drug Delivery Systems : DPPC is commonly used to create liposomes for drug delivery. These liposomes can encapsulate therapeutic agents, enhancing their stability and bioavailability .
- Case Study : A study demonstrated that incorporating DPPC into poly(lactic-co-glycolic acid) microspheres altered their surface chemistry, improving interaction with phagocytic cells and potentially enhancing drug delivery efficacy .
Application | Description |
---|---|
Drug Delivery | Used to form liposomes for encapsulating therapeutic agents |
Surface Chemistry Modification | Alters microsphere properties for better interaction with cells |
Pharmaceutical Formulations
DPPC's properties make it an ideal candidate for various pharmaceutical formulations beyond surfactants.
Inflammatory Response Modulation
- Immune System Interaction : Research indicates that DPPC can modulate inflammatory responses by influencing leukocyte activity in the lungs. This modulation may help mitigate excessive inflammation during respiratory infections .
- Experimental Findings : In vitro studies showed that preincubation with DPPC inhibited oxidative responses in human monocytes, suggesting potential therapeutic applications in inflammatory lung diseases .
Synthetic Surfactant Development
- Combination Therapies : Current synthetic surfactants often combine DPPC with other lipids and proteins to enhance efficacy against RDS. The optimal ratio of DPPC is critical for maximizing surfactant activity .
Advanced Research Techniques
DPPC is also employed in advanced research methodologies such as Atomic Force Microscopy (AFM) to study membrane dynamics.
Membrane Studies
特性
IUPAC Name |
2,3-di(hexadecanoyloxy)propyl 2-(trimethylazaniumyl)ethyl phosphate | |
---|---|---|
Source | PubChem | |
URL | https://pubchem.ncbi.nlm.nih.gov | |
Description | Data deposited in or computed by PubChem | |
InChI |
InChI=1S/C40H80NO8P/c1-6-8-10-12-14-16-18-20-22-24-26-28-30-32-39(42)46-36-38(37-48-50(44,45)47-35-34-41(3,4)5)49-40(43)33-31-29-27-25-23-21-19-17-15-13-11-9-7-2/h38H,6-37H2,1-5H3 | |
Source | PubChem | |
URL | https://pubchem.ncbi.nlm.nih.gov | |
Description | Data deposited in or computed by PubChem | |
InChI Key |
KILNVBDSWZSGLL-UHFFFAOYSA-N | |
Source | PubChem | |
URL | https://pubchem.ncbi.nlm.nih.gov | |
Description | Data deposited in or computed by PubChem | |
Canonical SMILES |
CCCCCCCCCCCCCCCC(=O)OCC(COP(=O)([O-])OCC[N+](C)(C)C)OC(=O)CCCCCCCCCCCCCCC | |
Source | PubChem | |
URL | https://pubchem.ncbi.nlm.nih.gov | |
Description | Data deposited in or computed by PubChem | |
Molecular Formula |
C40H80NO8P | |
Source | PubChem | |
URL | https://pubchem.ncbi.nlm.nih.gov | |
Description | Data deposited in or computed by PubChem | |
DSSTOX Substance ID |
DTXSID50910226 | |
Record name | 2,3-Bis(hexadecanoyloxy)propyl 2-(trimethylazaniumyl)ethyl phosphate | |
Source | EPA DSSTox | |
URL | https://comptox.epa.gov/dashboard/DTXSID50910226 | |
Description | DSSTox provides a high quality public chemistry resource for supporting improved predictive toxicology. | |
Molecular Weight |
734.0 g/mol | |
Source | PubChem | |
URL | https://pubchem.ncbi.nlm.nih.gov | |
Description | Data deposited in or computed by PubChem | |
Physical Description |
White powder; [Aldrich MSDS] | |
Record name | 1,2-Dipalmitoylphosphatidylcholine | |
Source | Haz-Map, Information on Hazardous Chemicals and Occupational Diseases | |
URL | https://haz-map.com/Agents/10537 | |
Description | Haz-Map® is an occupational health database designed for health and safety professionals and for consumers seeking information about the adverse effects of workplace exposures to chemical and biological agents. | |
Explanation | Copyright (c) 2022 Haz-Map(R). All rights reserved. Unless otherwise indicated, all materials from Haz-Map are copyrighted by Haz-Map(R). No part of these materials, either text or image may be used for any purpose other than for personal use. Therefore, reproduction, modification, storage in a retrieval system or retransmission, in any form or by any means, electronic, mechanical or otherwise, for reasons other than personal use, is strictly prohibited without prior written permission. | |
CAS No. |
2644-64-6 | |
Record name | 1,2-Dipalmitoylphosphatidylcholine | |
Source | CAS Common Chemistry | |
URL | https://commonchemistry.cas.org/detail?cas_rn=2644-64-6 | |
Description | CAS Common Chemistry is an open community resource for accessing chemical information. Nearly 500,000 chemical substances from CAS REGISTRY cover areas of community interest, including common and frequently regulated chemicals, and those relevant to high school and undergraduate chemistry classes. This chemical information, curated by our expert scientists, is provided in alignment with our mission as a division of the American Chemical Society. | |
Explanation | The data from CAS Common Chemistry is provided under a CC-BY-NC 4.0 license, unless otherwise stated. | |
Record name | 1,2-Dipalmitoylphosphatidylcholine | |
Source | ChemIDplus | |
URL | https://pubchem.ncbi.nlm.nih.gov/substance/?source=chemidplus&sourceid=0002644646 | |
Description | ChemIDplus is a free, web search system that provides access to the structure and nomenclature authority files used for the identification of chemical substances cited in National Library of Medicine (NLM) databases, including the TOXNET system. | |
Record name | 2,3-Bis(hexadecanoyloxy)propyl 2-(trimethylazaniumyl)ethyl phosphate | |
Source | EPA DSSTox | |
URL | https://comptox.epa.gov/dashboard/DTXSID50910226 | |
Description | DSSTox provides a high quality public chemistry resource for supporting improved predictive toxicology. | |
Record name | (4-oxido-10-oxo-7-palmitoyl-3,5,9-trioxa-4-phosphapentacosyl)trimethylammonium 4-oxide | |
Source | European Chemicals Agency (ECHA) | |
URL | https://echa.europa.eu/substance-information/-/substanceinfo/100.018.322 | |
Description | The European Chemicals Agency (ECHA) is an agency of the European Union which is the driving force among regulatory authorities in implementing the EU's groundbreaking chemicals legislation for the benefit of human health and the environment as well as for innovation and competitiveness. | |
Explanation | Use of the information, documents and data from the ECHA website is subject to the terms and conditions of this Legal Notice, and subject to other binding limitations provided for under applicable law, the information, documents and data made available on the ECHA website may be reproduced, distributed and/or used, totally or in part, for non-commercial purposes provided that ECHA is acknowledged as the source: "Source: European Chemicals Agency, http://echa.europa.eu/". Such acknowledgement must be included in each copy of the material. ECHA permits and encourages organisations and individuals to create links to the ECHA website under the following cumulative conditions: Links can only be made to webpages that provide a link to the Legal Notice page. | |
Record name | DIPALMITOYLPHOSPHATIDYLCHOLINE, DL- | |
Source | FDA Global Substance Registration System (GSRS) | |
URL | https://gsrs.ncats.nih.gov/ginas/app/beta/substances/2W15RT5V7V | |
Description | The FDA Global Substance Registration System (GSRS) enables the efficient and accurate exchange of information on what substances are in regulated products. Instead of relying on names, which vary across regulatory domains, countries, and regions, the GSRS knowledge base makes it possible for substances to be defined by standardized, scientific descriptions. | |
Explanation | Unless otherwise noted, the contents of the FDA website (www.fda.gov), both text and graphics, are not copyrighted. They are in the public domain and may be republished, reprinted and otherwise used freely by anyone without the need to obtain permission from FDA. Credit to the U.S. Food and Drug Administration as the source is appreciated but not required. | |
Retrosynthesis Analysis
AI-Powered Synthesis Planning: Our tool employs the Template_relevance Pistachio, Template_relevance Bkms_metabolic, Template_relevance Pistachio_ringbreaker, Template_relevance Reaxys, Template_relevance Reaxys_biocatalysis model, leveraging a vast database of chemical reactions to predict feasible synthetic routes.
One-Step Synthesis Focus: Specifically designed for one-step synthesis, it provides concise and direct routes for your target compounds, streamlining the synthesis process.
Accurate Predictions: Utilizing the extensive PISTACHIO, BKMS_METABOLIC, PISTACHIO_RINGBREAKER, REAXYS, REAXYS_BIOCATALYSIS database, our tool offers high-accuracy predictions, reflecting the latest in chemical research and data.
Strategy Settings
Precursor scoring | Relevance Heuristic |
---|---|
Min. plausibility | 0.01 |
Model | Template_relevance |
Template Set | Pistachio/Bkms_metabolic/Pistachio_ringbreaker/Reaxys/Reaxys_biocatalysis |
Top-N result to add to graph | 6 |
Feasible Synthetic Routes
Q1: Can infrared (IR) spectroscopy provide information about DPPC structure?
A2: Yes, IR spectroscopy is a powerful tool for studying DPPC. The methylene (CH2) stretching vibrations are sensitive to the conformation of the DPPC acyl chains. The frequencies of these vibrations shift depending on whether the chains are in an ordered, all-trans conformation or a disordered state containing gauche conformers. [, ]
Q2: How can we quantify the conformational disorder in DPPC acyl chains using IR?
A3: The relative intensities of specific CD2 rocking modes in the IR spectrum can be used to quantify one-, two-, and three-bond conformational disorder in DPPC. This method allows for a detailed analysis of acyl chain packing and the effects of additives like cholesterol. []
Q3: Does the presence of cholesterol affect the behavior of DPPC bilayers?
A5: Yes, cholesterol significantly influences DPPC bilayer properties. It can both fluidize the membrane at lower concentrations and condense it at higher concentrations, impacting the area compressibility modulus and overall mechanical behavior of the bilayer. []
Q4: How does ethanol impact DPPC bilayers containing sterols?
A6: Ethanol can disrupt lipid-sterol interactions in DPPC bilayers, leading to a decrease in the area compressibility modulus and making the membrane more prone to interdigitation. This effect is important for understanding the impact of ethanol on cell membranes, particularly in yeast fermentations. []
Q5: How does DPPC interact with the pulmonary surfactant protein SP-A?
A7: SP-A interacts with DPPC in the presence of calcium ions, forming a ternary complex. This interaction enhances the thermal stability of SP-A and modifies the DPPC acyl chain tilt angle in monolayer films, suggesting a specific mode of interaction at the air/water interface. []
Q6: Can we use fluorescence techniques to study the interaction of proteins with DPPC?
A8: Yes, fluorescence energy transfer studies have been used to investigate the self-association of the pulmonary surfactant protein SP-C in DPPC vesicles. This technique revealed that SP-C self-association is dependent on the presence of gel-phase lipids and provides insights into its aggregation state in pulmonary surfactant. []
Q7: How does the structure of a peptide influence its interaction with DPPC monolayers?
A9: The presence or absence of specific structural motifs in peptides can significantly alter their interactions with DPPC monolayers. For example, deleting the helical domain in intestinal fatty acid-binding protein (IFABP) reduces its interaction with DPPC, as evidenced by slower domain formation and reduced lipid acyl chain ordering. []
Q8: How does the lipid environment impact the activity of enzymes like phospholipase D (PLD)?
A10: The activity of PLD, which hydrolyzes DPPC to 1,2-dipalmitoylphosphatidic acid (DPPA), is influenced by the structural organization of DPPC. PLD exhibits maximum activity in the more disordered liquid-expanded phase of DPPC monolayers. The presence of the hydrolysis product, DPPA, can further modulate PLD activity by inducing structural changes in DPPC-rich domains. []
Q9: Can DPPC be used in drug delivery systems?
A11: Yes, DPPC is a common component of liposomes, which are spherical vesicles formed by a lipid bilayer. Liposomes can encapsulate drugs and deliver them to specific targets in the body. [, ] The choice of lipid, including DPPC, influences liposomal properties like drug loading, cellular uptake, and stability. []
Q10: How can computational chemistry contribute to our understanding of DPPC?
A13: Molecular dynamics simulations are valuable tools to study DPPC bilayer behavior. These simulations can provide detailed insights into the molecular mechanisms of DPPC interactions with drugs, peptides, and other lipids, aiding in the rational design of drug delivery systems and other applications. [, ]
試験管内研究製品の免責事項と情報
BenchChemで提示されるすべての記事および製品情報は、情報提供を目的としています。BenchChemで購入可能な製品は、生体外研究のために特別に設計されています。生体外研究は、ラテン語の "in glass" に由来し、生物体の外で行われる実験を指します。これらの製品は医薬品または薬として分類されておらず、FDAから任何の医療状態、病気、または疾患の予防、治療、または治癒のために承認されていません。これらの製品を人間または動物に体内に導入する形態は、法律により厳格に禁止されています。これらのガイドラインに従うことは、研究と実験において法的および倫理的な基準の遵守を確実にするために重要です。